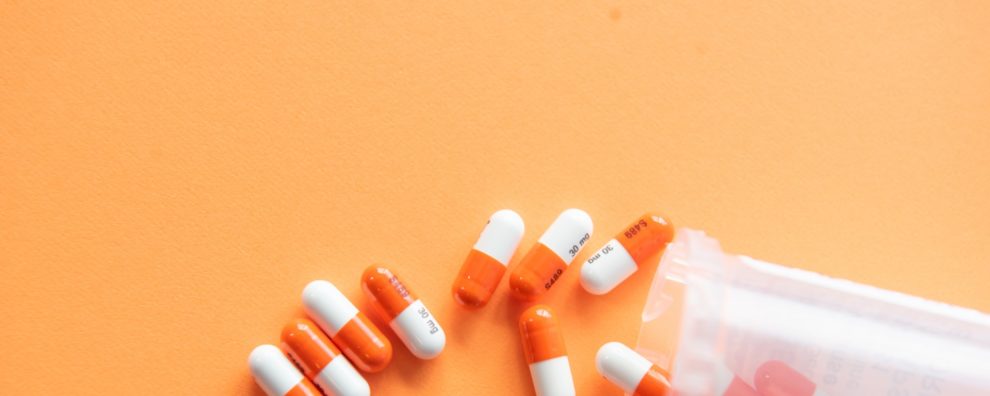
Everything you need to know about the generation of antibody-drug conjugates (ADCs)
Antibody-drug conjugates (ADCs) consist of three main elements: an antibody carrier, a cleavable or non-cleavable linker, and a bioactive payload. In this article, we discuss how each of these elements contributes to the therapeutic efficiency of ADCs, provide examples of the most commonly used compounds, and review the most important trends.
- What are antibody-drug conjugates (ADCs)?
- Structure of ADCs
- Antibody design and conjugation methods
- Target selection for efficient ADC design
- Antibody selection for ADC development
- Antibody conjugation methods for ADC production
- Linker chemistry for ADC development
- Cleavable versus non-cleavable linkers
- Challenges and advantages of both types of linker chemistry
- Major and emerging payloads of ADCs
What are antibody-drug conjugates (ADCs)?
Antibody-drug conjugates (ADCs) are an emerging and highly promising class of targeted therapies that combine the specificity and long half-life of antibodies with the high potency of small drugs. This combined form of therapy is used to deliver highly toxic payloads to specific cells or tissues, thus minimizing systemic exposure and reducing adverse side effects of otherwise aggressive forms of treatment.
The synergistic relationship between antibodies and drugs allows the use of payloads that are too toxic for systemic administration, such as the ones used in the treatment of cancer. All ADCs approved to date and many in clinical trials were designed to tackle different types of cancer, especially those that are resistant (relapsed or refractory) to more conventional forms of therapy.
The major mechanism of action of anti-cancer ADCs relies on the principle of internalization or targeted drug release. For this reason, great importance has been given to the development of responsive and robust linkers. It is the linker that determines the physiological conditions where the drug release takes place. Thus, linker chemistry is one of the most active subfields of research when it comes to ADC development.
Due to the great investment made in the development of strong and responsive linkers, ADC therapies have become progressively safer in recent years. In turn, this advance has allowed the development of increasingly toxic drugs and granted researchers the opportunity to explore new mechanisms of action and tackle new diseases.
Structure of ADCs
Antibody-drug conjugates (ADCs) consist of three main elements:
- A target-specific antibody carrier (monoclonal, bispecific, and even antibody fragments have been used for ADC applications)
- A cleavable or non-cleavable linker
- A cytotoxic payload (small drugs, protein toxins, enzymes, and antibiotics, among others, have been used for ADC development)
Typically, antibodies carry more than one molecule of a payload, allowing the accumulation of high concentrations of cytotoxic agents at the targeted site. The number of drug molecules and their distribution on the surface of the carrier differs significantly depending on the intended application of ADC, the potency of the drug, and the number of sites available for conjugation on the surface of the antibody carrier.
Antibody design and conjugation methods
Target selection for efficient ADC design
Target selection can dictate the success or failure of ADC therapies. Several factors need to be considered during this stage:
- Specificity: antibody carriers should strictly bind to surface-bound disease markers. These markers should either be absent from other tissues, expressed at low levels in vital tissues, or expressed at moderate levels in expendable or rapid regenerating tissues.
- Heterogeneity: diseases such as cancer are known for their high inter-patient variability. For this reason, comprehensive knowledge of target heterogeneity is vital to designing ADCs for each specific subgroup of patients.
- Abundance: independently of the mechanism of action (internalizing or non-internalizing) the target should be sufficiently abundant, easily accessible, and rapidly recycled by the cells in order to allow the accumulation of high levels of the cytotoxic agent.
Antibody selection for ADC development
The major mechanism of action of ADCs depends of three processes: internalization of the antibody-drug complex; efficient cellular tracking; and subsequent lysosomal degradation, leading to intracellular drug release. These events are controlled by the properties of the antibody carrier. As a general rule, strong antibody affinity and rapid internalization rates lead to highly efficient ADC therapies, but clinical research has shown us this correlation is not always linear.
For instance, some slow internalizing ADCs such as Kadcyla™ have found great success in the clinic. Moreover, internalizing ADCs are known to have limited efficiency against solid tumors where diffusion rates are slow due to the antigen barrier imposed by peripheral cancer cells. For this reason, it is increasingly recognized among the scientific community that efficient ADC therapies can act via more than a single mechanism.
Moreover, the development of non-internalizing ADCs able to rapidly release their toxic payloads in the extracellular space may widen the therapeutic window and allow the application of ADCs to the treatment of other medical conditions.
Antibody conjugation methods for ADC production
Antibody conjugation methods can be classified as chemical or enzymatic. The chemical conjugation of ADCs depends on the presence and distribution of reactive functional groups on the surface of the antibody. A few examples of the methods that use this type of conjugation include those that leverage lysine (reactive amine groups), cysteine (reaction between cysteine residues and thiol groups inserted in the payload), and tyrosine residues, among others.
In contrast, enzymatic methods rely on the use of enzymes to modify the antibody in a site or sequence-specific manner. The most widely used enzymes in bioconjugation include sortase A (cleaves the threonine-glycine bond and attaches an oligoglycine molecule) and the microbial transglutaminase (modifies conserved glycosylation sites).
One of the most exciting and important trends in antibody design and conjugation is the use of click chemistry for ADC development, also known as bioorthogonal chemistry. These methods rely on very rapid and spontaneous reactions between groups that are not usually found in biomolecules. The term click is used to illustrate the “fusion” between two complementary molecules that can only react with each other. The advantage of this type of conjugation is that is extremely cost-effective, rapid, selective, and it allows better control over the number and distribution of conjugation sites because it does not depend on natural residues present on the antibody carrier.
Linker chemistry for ADC development
Linkers can be defined as the interface between biology and chemistry serving as the bridge that links antibodies and drugs in ADCs. Linker chemistry in ADCs dictates the stability, safety, therapeutic potency and window, and efficacy of ADCs. They also determine the mechanism of action of these complex therapeutics, warranting the need for extensive analysis of these drugs before they can be considered ready for clinical studies or trials.
Linker technology can be defined as cleavable or non-cleavable, each catering to different mechanisms of action. Independently of their class, linkers typically consist of two major domains: a payload-binding domain and an antibody-binding domain.
Cleavable versus non-cleavable linkers
Linker chemistry can be divided into cleavable or non-cleavable. However, the vast majority of ADCs in the clinic or in active development can be classified as chemically-labile linkers. These linkers incorporate chemical triggers allowing them to degrade in response to specific signals or conditions. In contrast, non-cleavable linkers are designed to be fully and stably integrated into the payload. In this case, the payload-linker complex is only released upon the degradation of the ADC molecule by lysosomal enzymes.
- Cleavable linkers
- Chemically-labile linkers: hydrazones (susceptible to low pH values) and disulfide (glutathione sensitive) linkers
- Enzymatically-labile linkers: peptide (e.g., valine and citrulline susceptible to intracellular proteases), β-glucuronide, β-galactoside, and PEGylated linkers, among others
- Non-cleavable linkers: thioether linkers (e.g., maleimidomethyl cyclohexane-1-carboxylate, MCC linker)
Challenges and advantages of both types of linker chemistry
Generally, cleavable linkers are easier to develop given that they are compatible with a broader range of payloads. Because these linkers are cleaved from the payload, often without leaving residual components, they do not interfere with the drug’s bioactivity. This chemistry enhances the efficiency of drug release by ADCs. Moreover, it is better suited to target solid tumors because it allows the release of payloads in the extracellular space, thus leading to a more efficient uptake by cancer cells that might be devoid of the target antigen (bystander effect). One of the most important drawbacks of these linkers is precisely their sensitivity to chemical stimuli, which might result in the release of payloads off-site and subsequently lead to higher systemic toxicity.
Non-cleavable linkers attempt to overcome these stability issues by ensuring that the drug is only released in the intracellular compartment. This chemistry results in safer and more stable ADCs that can be conjugated to extremely toxic cargoes. However, because these linkers are never cleaved from the payload, they are known to modify its structure, often leading to considerable changes in drug potency. For this reason, payload-drug pairs need to be extensively tested in vitro before developers can estimate their toxicity in vivo. At the moment, very few classes of payloads have been successfully conjugated with non-cleavable linkers.
The vast majority of ADCs in the clinic incorporate peptide linkers such as valine-citrulline (Val-Vit) that are degraded by lysosomal proteases such as cathepsin B. This protease is known to leak to the extracellular compartment in necrotic tissues, resulting in extracellular cleavage of ADCs at tumor sites, especially useful when targeting solid tumors.
Recently, there is a growing interest in the development of alternative linkers such as those containing hydrophilic motifs (e.g., β-glucuronic acid motifs). However, this new generation of linkers has yet to reach its full technological maturity.
Major and emerging payloads of ADCs
Payloads are the bioactive component of ADCs. Most payloads used in ADC generation are too toxic to be administered systemically, for this reason, they can only be used in targeted therapeutic applications. Most cytotoxic agents that have found great clinical success exhibit high activity at the nanomolar or picomolar range. Moreover, these agents must be fairly soluble, non-immunogenic, and possess reactive sites for bioconjugation with a suitable linker.
The majority of payloads used in FDA-approved ADCs can be classified as tubulin inhibitors or DNA-damaging agents. The first class acts on tubulin, leading to the arrest of the growth cycle and subsequent cell death by apoptosis. In contrast, the second class is independent of the growth stage of the cell, alternatively acting as molecular scissors that cleave the DNA leading to cell death.
Due to the progressive increase of the safety of linker chemistry, researchers are actively exploring alternative types of payloads including pyrrolobenzodiazepines (PBD) dimers, which have led to very promising results in short periods. Moreover, constructs that employ the use of payloads such as protein toxins, enzymes, and antibiotics are growing fields of interest with vast potential.
Payload class | Mechanism of action | Examples |
---|---|---|
Auristatins | Tubulin polymerase inhibitor | Monomethyl auristatin E (MMAE) and Monomethyl auristatin F (MMAF) |
Maytansinoids | Tubulin depolymerization | Maytansine derivates (DM1 and DM4) |
Calicheamicins | DNA cleavage | Calicheamicin γ1 |
Duocarymycins | DNA minor groove alkylating agent | CC-1065 and duocarmycin SA |
Pyrrolobenzodiazepines (PBD) dimers | DNA minor groove cross-linker | PBD derivates |
Amatoxins | RNA polymerase II inhibitor | α-Amanitin |
Protein toxins | Several | Pseudomonas exotoxin (PE) and diphtheria toxin (DT) |
Antibiotics | Several | 4-dimethylamino piperidino-hydroxybenzoxazino rifamycin (dmDNA31) |
Enzymes | Several | β-glucuronidase, urease, among others |
You could also be interested in: