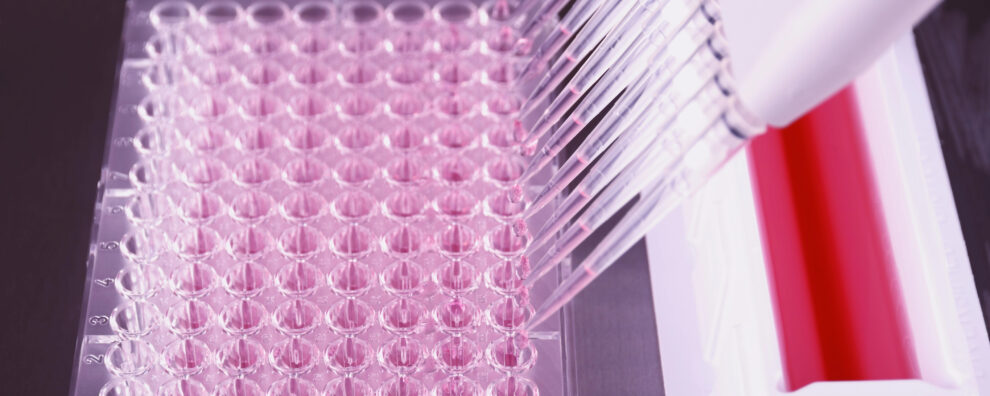
Top Tips for Extracting High-Performing Antibodies from Phage Display
Antibody discovery is a cornerstone of modern biomedical research and therapeutic development. Monoclonal antibodies (mAbs) have revolutionized the treatment of a wide range of diseases, including cancers, autoimmune disorders, and infectious diseases. Their high specificity and ability to bind to unique targets make them invaluable tools in both diagnostics and therapeutics. As the demand for new and more effective antibodies continues to grow, researchers are constantly seeking innovative methods to streamline the discovery process and enhance antibody performance. One such innovative method is Phage Display technology. Introduced in the mid-1980s, Phage Display has become a powerful tool for antibody discovery and engineering. This technique allows for the presentation of vast libraries of antibody variants on the surface of bacteriophages (viruses that infect bacteria). By exposing these libraries to target antigens, researchers can selectively enrich for antibodies with high affinity and specificity. Phage Display not only accelerates the antibody discovery process but also enables the generation of antibodies against challenging targets that may be difficult to address using traditional methods such as Hybridoma technology. Given the potential of Phage Display to produce high-performing antibodies, it is essential to optimize each step of the process to maximize success. This blog aims to provide an exhaustive guide filled with actionable tips and best practices for researchers and scientists looking to enhance their Phage Display outcomes. By following these guidelines, you can improve the quality and performance of your antibodies, ultimately advancing your research and therapeutic projects. In this guide, we will cover crucial aspects of the Phage Display process, including optimizing library diversity, effective selection and screening strategies, techniques for enhancing antibody affinity, troubleshooting common issues, and thorough validation and characterization methods. Whether you are new to Phage Display or looking to refine your existing protocols, this comprehensive resource will help you achieve the best possible results.
- What is Phage Display?
- What are Phage Display libraries?
- Optimizing Library Diversity
- Designing a Diverse Phage Library
- Using Synthetic and Natural Sources
- Advantages of Using Synthetic Libraries for Targeted Diversity and Natural Libraries for Broader Diversity
- Effective Selection and Screening Strategies
- Biopanning Process Optimization
- Increasing Selection Stringency
- Innovative Screening Methods
- Enhancing Antibody Affinity
- Affinity Maturation Techniques
- Structural Analysis and Computational Design
- Troubleshooting and Avoiding Common Pitfalls
- Common Challenges and Solutions
- Maintaining Phage Library Integrity
- Comprehensive Validation and Characterization
- inding Assays for Validation
- Cross-Reactivity and Specificity Testing
- Conclusion
- References
What is Phage Display?
Phage Display is a powerful technique that involves displaying peptides or proteins, such as antibodies, on the surface of bacteriophages. By creating a vast library of phage particles, each displaying a unique antibody variant, researchers can screen for those that bind with high affinity to a specific target antigen. This method allows for rapid identification and optimization of antibodies, making it a cornerstone in antibody discovery and engineering.
For a deeper understanding of Phage Display technology, including its history, methodology, and applications, please visit our Phage Display Technology Guide.
What are Phage Display libraries?
A phage library is a collection of bacteriophages that have been genetically engineered to display a variety of antibody fragments on their surfaces. The diversity of this library is crucial as it determines the range of antibodies available for screening and selection. Optimizing the diversity of your phage library is essential to ensure the successful identification of high-affinity antibodies.
Optimizing Library Diversity
Designing a Diverse Phage Library
To fully harness the potential of Phage Display, it is imperative to start with a highly diverse phage library. This diversity ensures a broad range of antibody variants, increasing the likelihood of identifying high-affinity binders. Below are key strategies to design a diverse phage library:
- Importance of Diversity in the Initial Phage Library
Capturing a Wide Range of Antibodies: The initial diversity of the phage library significantly influences the success of the selection process. A diverse library encompasses a wide array of antibody fragments, which enhances the probability of identifying high-affinity binders specific to different epitopes on the target antigen.
Enhancing Screening Success: High diversity ensures a comprehensive representation of possible antigen-binding sites, which is crucial for identifying optimal binders during the screening process.
- Strategies for Incorporating Diverse Genetic Elements
Incorporating diverse genetic elements into your phage library is crucial for maximizing the potential to discover high-affinity antibodies. Here are scientifically-backed strategies to achieve this:
Source Selection
Utilize Genetic Material from Various Origins: Enhancing genetic diversity can be achieved by incorporating genetic sequences from a wide range of sources. For example, human, mouse, and other species can provide a broad spectrum of antibody fragments. Additionally, synthetic libraries, designed through computational methods, can introduce unique sequences that mimic natural diversity. By combining these sources, the library can display a wide array of binding properties, increasing the chances of identifying high-affinity antibodies.
Leverage Immunized and Non-Immunized Sources: Libraries derived from immunized animals tend to have higher affinity antibodies due to prior antigen exposure. However, incorporating sequences from non-immunized sources can introduce novel binding motifs not influenced by immune selection, thus broadening the diversity of potential binders.
Random Mutagenesis
Error-Prone PCR: This technique involves the deliberate introduction of mutations into the DNA sequence during amplification. By using conditions that reduce the fidelity of DNA polymerase, a variety of mutations can be introduced, generating a diverse array of antibody variants. This mimics the natural process of somatic hypermutation, which is crucial for antibody diversification in vivo.
DNA Shuffling: DNA shuffling involves the fragmentation and reassembly of a pool of related DNA sequences, creating new combinations of genetic material. This method can rapidly generate a diverse library by recombining functional domains from different antibodies, enhancing the potential to identify novel high-affinity binders.
Recombinant Techniques
Combining VH and VL Chains from Different Sources: By using recombinant DNA technology to combine variable heavy (VH) and variable light (VL) chains from different antibodies, you can create unique combinations that enhance the diversity of the library. This method allows for the exploration of new antigen-binding sites that might not be present in natural pairings, thereby increasing the likelihood of finding high-affinity interactions.
Incorporating CDR Diversity
Synthetic CDR Libraries: Design libraries that specifically vary the CDR regions while keeping the framework regions constant. This targeted approach focuses diversity on the antigen-binding regions, which are critical for antibody-antigen interactions. Synthetic CDR libraries can be designed to include a wide range of possible amino acid sequences, enhancing the overall diversity of the library.
Using Synthetic and Natural Sources
Combining Synthetic and Natural Antibody Sequences to Enhance Diversity
Combining synthetic and natural antibody sequences in phage libraries is a powerful strategy to maximize the diversity and functionality of the antibodies discovered. This hybrid approach leverages the strengths of both sources to create a robust and versatile library.
- Synthetic Libraries
Designed Diversity: Synthetic libraries are engineered to include a wide range of genetic variations, particularly in the complementarity-determining regions (CDRs). This allows for precise control over the diversity and enables the targeting of specific antigen epitopes that are known to be difficult for natural antibodies to recognize.
Customizable Features: Synthetic sequences can be designed to incorporate specific features, such as increased stability, reduced immunogenicity, or enhanced binding affinity. This customization is particularly useful for therapeutic antibody development where specific characteristics are desirable.
- Natural Libraries
Broad Diversity from Immune Responses: Natural libraries are derived from the B cells of immunized or non-immunized animals or humans. These libraries reflect the natural diversity generated through immune responses, providing a broad repertoire of antibodies that have undergone natural selection processes.
Functional Affinity Maturation: Antibodies from natural libraries have typically undergone affinity maturation in vivo, resulting in high-affinity binders. This process can yield antibodies with higher initial affinities and specificities compared to those from synthetic libraries.
Advantages of Using Synthetic Libraries for Targeted Diversity and Natural Libraries for Broader Diversity
- Synthetic Libraries
Precision and Control: Synthetic libraries allow for precise control over the genetic diversity introduced into the library. Researchers can design specific mutations or variations to explore particular binding properties or to enhance the binding affinity for known antigens.
High Repeatability: Synthetic libraries can be consistently reproduced, ensuring that the same diversity is available for repeated experiments. This repeatability is crucial for validating results and for large-scale antibody production.
- Natural Libraries
Comprehensive Immune Repertoire: Natural libraries encompass the entire range of antibodies produced by an organism’s immune system. This broad diversity includes rare and unique antibodies that might not be present in synthetic libraries, enhancing the chances of discovering novel binders.
Real-World Selection Pressure: The antibodies in natural libraries have been shaped by real-world selection pressures, including exposure to pathogens. This natural selection can produce antibodies with unique binding characteristics and higher affinities.
Effective Selection and Screening Strategies
Optimizing the selection and screening processes in Phage Display is crucial for isolating high-affinity antibodies. Here are detailed steps and tips to enhance each phase of the process:
Biopanning Process Optimization
- Detailed Steps for Biopanning:
Binding: During the binding phase, the phage library is incubated with the target antigen. This allows phages displaying antibodies that bind to the antigen to be captured. Ensure the antigen is immobilized on a solid support, such as a microtiter plate, to facilitate binding. The binding step can be optimized by adjusting the pH and ionic strength of the binding buffer to enhance the interaction between the phage-displayed antibodies and the antigen.
Washing: After binding, the non-specific phages are removed through a series of washes. The stringency of the washing steps is critical in eliminating weak or non-specific binders. Use buffers that disrupt non-specific interactions without denaturing the target or the phage particles. Increasing the volume and number of washes can help improve the selection of high-affinity binders.
Elution: The bound phages are then eluted, typically using an acidic or basic buffer, competitive ligand, or enzymatic cleavage. Each method has its advantages depending on the nature of the antigen and antibody interaction. For example, using a low pH buffer can elute phages by disrupting ionic interactions, while competitive elution with an excess of soluble antigen can specifically displace phages bound through high-affinity interactions.
- Tips for Adjusting Panning Conditions to Improve Specificity and Affinity of Selected Antibodies
Optimize Antigen Presentation: Ensure that the antigen is presented in a form that closely mimics its natural conformation. This can be achieved by using full-length proteins or relevant protein fragments. Surface coating methods such as biotinylation followed by binding to streptavidin-coated surfaces can improve antigen presentation.
Control Incubation Time and Temperature: Adjust the incubation time and temperature during the binding phase to enhance the interaction between the phage and the antigen. Lower temperatures can reduce non-specific interactions, while longer incubation times can allow for more complete binding.
Use of Blocking Agents: Incorporate blocking agents, such as BSA or casein, during the binding and washing steps to reduce background noise from non-specific binding. This helps in improving the signal-to-noise ratio and the overall specificity of the selected antibodies.
- Importance of Maintaining Consistent and Stringent Washing Steps to Remove Non-Specific Binders
Consistency in Washing Protocols: Use the same washing protocol throughout the panning rounds to ensure comparability of results. Varying the washing stringency inconsistently can lead to the selection of phages with varying affinities, complicating the selection process.
Increasing Washing Stringency: Gradually increase the stringency of washing with each panning round. This can be done by increasing the wash volume, number of washes, or using more stringent buffers. Consistent and stringent washing helps in enriching the library with high-affinity binders by removing weakly bound and non-specific phages.
Increasing Selection Stringency
- Gradual Increase in Selection Stringency Across Multiple Panning Rounds to Enrich High-Affinity Binders
Initial Rounds with Low Stringency: Begin with low stringency conditions to capture a broad range of potential binders. This helps to ensure that you do not miss any low-affinity binders that might evolve into high-affinity binders through subsequent rounds. Initial rounds often involve fewer washes and lower concentrations of washing agents.
Incremental Stringency Increase: Gradually increase the stringency of the selection process across multiple panning rounds. This can involve reducing the antigen concentration, increasing the number of washes, or using harsher washing buffers. This approach selectively enriches for high-affinity binders by applying increasing selection pressure.
Monitor Binder Enrichment: Use ELISA or another binding assay to monitor the enrichment of high-affinity binders after each panning round. This helps in determining the optimal number of panning rounds needed. Observing the binding signal increase over successive rounds indicates successful enrichment of high-affinity phages.
- Techniques for Fine-Tuning the Stringency
Reducing Antigen Concentration: Lowering the antigen concentration in successive panning rounds can help in selecting phages with higher binding affinity. This selective pressure ensures that only the phages with the strongest interactions with the antigen are retained.
Increasing Wash Times: Extend the duration of washing steps to enhance the removal of weakly bound phages. This technique ensures that only phages with strong binding interactions remain attached to the antigen. Adjusting the ionic strength and pH of the washing buffer can also help in fine-tuning the stringency of the selection process.
Innovative Screening Methods
- Use of Next-Generation Sequencing (NGS) to Analyze the Diversity and Evolution of Phage Libraries
High-Throughput Sequencing: Implement NGS to sequence the antibody fragments displayed on the phages. This provides a comprehensive view of the diversity present in the library and helps in identifying dominant clones that emerge through the selection process. NGS allows for the identification of even rare clones that might possess high-affinity binding properties.
Evolutionary Tracking: Track the evolution of antibody sequences across different panning rounds to understand how selection pressures influence the diversity and affinity of the phage library. Analyzing sequence data from different rounds can reveal the enrichment patterns of specific clones and help in optimizing the selection process.
- High-Throughput Screening Techniques to Quickly Identify High-Affinity Clones
Fluorescence-Activated Cell Sorting (FACS): Use FACS to sort phages based on the fluorescence intensity of labeled antigens bound to phage-displayed antibodies. This technique allows for the rapid and accurate identification of high-affinity binders. FACS can be combined with labeled antigens or secondary antibodies to selectively isolate high-affinity phage clones.
Surface Plasmon Resonance (SPR): Employ SPR to measure the binding kinetics and affinities of selected phages. SPR provides real-time analysis and is useful for ranking clones based on their binding characteristics. This method allows for the precise determination of kinetic parameters such as association and dissociation rates, which are critical for assessing the quality of the selected antibodies.
Enhancing Antibody Affinity
Affinity maturation is a critical step in optimizing antibodies for high-affinity binding to their target antigens. This process involves introducing variations and selecting the best-performing mutants to improve binding characteristics. Discover ProteoGenix’s Affinity Maturation service. Here are detailed strategies and techniques for enhancing antibody affinity:
Affinity Maturation Techniques
- Role of Mutagenesis in Introducing Variations for Affinity Improvement
Error-Prone PCR: This technique introduces random mutations throughout the antibody coding sequences during PCR amplification. By using conditions that lower the fidelity of DNA polymerase, a variety of mutations are generated, increasing the diversity of the antibody library. This mimics the natural somatic hypermutation process, allowing for the selection of antibodies with improved binding affinities.
DNA Shuffling: Also known as molecular breeding, DNA shuffling involves fragmenting and reassembling a pool of related DNA sequences. This recombination of antibody fragments can create new variants with enhanced properties by combining beneficial mutations from different parental sequences. DNA shuffling can be particularly effective in generating antibodies with improved binding affinities and specificities.
Site-Directed Mutagenesis: Targeted mutations are introduced at specific positions within the antibody sequence, particularly within the complementarity-determining regions (CDRs). This allows for precise modifications to the antibody binding site, facilitating the enhancement of affinity through rational design. Site-directed mutagenesis is useful for systematically exploring the impact of specific amino acid changes on binding.
- Strategies for Selecting Higher-Affinity Mutants
Competitive Elution: During the biopanning process, competitive elution can be used to preferentially elute phages displaying higher-affinity antibodies. This involves using a soluble form of the antigen to displace the bound phages. Phages with higher binding affinities are less likely to be displaced, allowing for their selective enrichment.
Off-Rate Screening: This technique involves incubating the phage-antigen complex for extended periods, followed by stringent washing to remove phages with faster dissociation rates. Phages that remain bound are likely to have slower off-rates and, therefore, higher affinities. Off-rate selection helps in identifying antibodies that not only bind tightly but also dissociate slowly from their target antigens.
Structural Analysis and Computational Design
- Using Structural Analysis and Computational Modeling to Predict and Enhance Antibody-Antigen Interactions
Structural Analysis: Determining the three-dimensional structure of antibody-antigen complexes using techniques like X-ray crystallography or cryo-electron microscopy provides insights into the binding interactions at the molecular level. This information can be used to identify key residues involved in binding and to design mutations that enhance affinity.
Computational Modeling: In silico methods, including molecular dynamics simulations and docking studies, can predict how antibodies interact with antigens. These models help in visualizing potential binding sites and conformational changes, guiding the design of antibodies with improved binding characteristics. Computational tools can also be used to screen large libraries of antibody variants quickly, identifying those with the best predicted affinities.
- Tools and Software Available for In Silico Affinity Maturation and Redesign
RosettaAntibody: This software suite includes tools for predicting antibody structures, modeling antibody-antigen interactions, and designing mutations to improve binding affinity. RosettaAntibody uses advanced algorithms to optimize antibody sequences and structures, making it a powerful tool for in silico affinity maturation.
PyMOL: A molecular visualization system that allows researchers to visualize and manipulate the structures of antibody-antigen complexes. PyMOL can be used to identify critical interactions and design mutations to enhance binding affinity. It is widely used for structural analysis and design in antibody engineering.
Maestro: An integrated platform that combines molecular modeling, simulation, and visualization tools. Maestro provides capabilities for docking studies, molecular dynamics simulations, and protein engineering, making it suitable for designing high-affinity antibodies.
Troubleshooting and Avoiding Common Pitfalls
In the Phage Display process, various challenges can arise that may affect the yield, affinity, and overall quality of the antibodies produced. Identifying and addressing these issues is crucial for successful antibody discovery. Below are detailed strategies for troubleshooting common problems and maintaining the integrity of your phage library.
Common Challenges and Solutions
- Addressing Issues like Low Phage Yield, Poor Binding Affinity, and High Background Noise
Low Phage Yield: Low phage yield can result from inefficient phage amplification or poor host cell health. Ensure that the bacterial host cells (commonly E. coli) are in the exponential growth phase and are not overgrown, as this can reduce transformation efficiency. Optimize the induction conditions for phage production, such as the concentration of the inducer (e.g., IPTG) and the duration of induction.
Poor Binding Affinity: This issue can stem from a lack of diversity in the phage library or suboptimal panning conditions. Enhance library diversity by incorporating various genetic elements and using mutagenesis techniques. Optimize panning conditions, such as adjusting the antigen concentration and incubation time, to improve the selection of high-affinity binders.
High Background Noise: High background noise can be caused by non-specific binding of phages to the assay surface or the target antigen. Use appropriate blocking agents, such as BSA or casein, during binding and washing steps to minimize non-specific interactions. Implement stringent washing steps to remove weakly bound phages and reduce background noise.
- Tips for Optimizing Phage Growth Conditions and Ensuring the Quality of the Phage Library
Optimizing Growth Conditions: Maintain optimal growth conditions for the bacterial host cells by using fresh media and maintaining the appropriate temperature (usually 37°C for E. coli). Monitor the optical density (OD600) of the culture to ensure that cells are harvested at the right growth phase.
Ensuring Library Quality: Regularly assess the quality of the phage library by sequencing a subset of the library to verify diversity. Monitor the phage titer to ensure that the library remains at a high concentration. Avoid repeated freeze-thaw cycles, which can reduce phage viability and library diversity.
Maintaining Phage Library Integrity
- Best Practices for Storing and Handling Phage Libraries to Prevent Degradation and Loss of Diversity
Storage Conditions: Store phage libraries at -80°C in a glycerol stock or as phage particles in a suitable buffer. Avoid frequent freeze-thaw cycles by aliquoting the library into smaller volumes. Ensure that the storage buffer contains cryoprotectants, such as glycerol, to maintain phage stability.
Handling Precautions: Handle phage libraries with care to avoid contamination. Use sterile techniques and avoid exposing the library to high temperatures or harsh conditions that could degrade the phage particles. When working with phage libraries, always use freshly prepared aliquots to ensure consistency.
- Regular Monitoring and Quality Checks to Ensure Library Integrity Over Time
Titer and Diversity Assessment: Periodically assess the titer of the phage library by plating serial dilutions on bacterial lawns and counting the resulting plaques. This helps in monitoring the concentration and viability of the phage particles. Additionally, sequence a random subset of the library to ensure that genetic diversity is maintained over time.
Functional Validation: Regularly validate the functionality of the phage library by performing panning experiments and assessing the enrichment of target-specific binders. This helps in identifying any issues with library performance early on and allows for corrective actions to be taken.
Comprehensive Validation and Characterization
Validation and characterization of antibodies obtained from Phage Display are critical steps to ensure their functionality and specificity. Detailed strategies and techniques for comprehensive validation are provided below:
inding Assays for Validation
- Techniques such as ELISA, SPR, and BLI for Assessing Binding Specificity and Affinity
Enzyme-Linked Immunosorbent Assay (ELISA): ELISA is a widely used technique for assessing the binding specificity and affinity of antibodies. It involves coating a microplate with the target antigen, incubating with the antibody, and detecting bound antibodies using an enzyme-linked secondary antibody that produces a colorimetric signal. This method is suitable for high-throughput screening and provides quantitative data on antibody-antigen interactions (Crowther, 2000).
Surface Plasmon Resonance (SPR): SPR is an optical technique that measures changes in the refractive index near the surface of a sensor chip to detect binding events in real-time. It provides detailed kinetic data, including association and dissociation rates, which are essential for understanding the binding affinity and specificity of antibodies. SPR can be used to compare the binding kinetics of different antibody variants and optimize their performance (Rich & Myszka, 2008).
Bio-Layer Interferometry (BLI): BLI uses interference patterns of light to measure binding events on the surface of a biosensor. Like SPR, it provides real-time kinetic data but with a simpler and more flexible setup. BLI is suitable for both high-throughput screening and detailed kinetic studies, making it a versatile tool for antibody characterization.
- Importance of Validating Binding Under Various Conditions to Ensure Robustness
Buffer Conditions: Test antibody binding in different buffer conditions to assess robustness. Variations in pH, ionic strength, and the presence of different salts or detergents can affect binding interactions. Ensuring that antibodies maintain their binding properties under various conditions is crucial for their practical application.
Temperature Stability: Evaluate the stability of antibody binding at different temperatures. This is particularly important for therapeutic antibodies that need to remain effective at physiological temperatures and during storage and transport.
Competitive Binding Assays: Perform competitive binding assays to assess the ability of antibodies to compete with known ligands or other antibodies for the same epitope. This helps in determining the relative affinity and specificity of the selected antibodies.
Cross-Reactivity and Specificity Testing
- Methods for Testing Cross-Reactivity with Related Antigens to Ensure Specificity
Cross-Reactivity ELISA: Use ELISA to test antibody binding against a panel of related antigens. This helps in identifying any cross-reactivity and ensures that the antibody is specific to the target antigen. Include structurally similar proteins and irrelevant antigens in the panel to comprehensively evaluate specificity.
Epitope Mapping: Perform epitope mapping to identify the specific regions on the antigen that the antibody binds to. Techniques such as peptide scanning, alanine scanning mutagenesis, and phage display of epitope libraries can provide detailed information on the binding epitope. Epitope mapping helps in understanding the specificity of the antibody and predicting potential cross-reactivity.
- Importance of Thorough Specificity Testing for Therapeutic and Diagnostic Applications
Therapeutic Applications: For therapeutic antibodies, ensuring high specificity is crucial to avoid off-target effects that can lead to adverse reactions. Specificity testing helps in minimizing the risk of cross-reactivity with human proteins, thereby enhancing the safety profile of the therapeutic antibody.
Diagnostic Applications: In diagnostics, high specificity is essential to avoid false positives and ensure accurate detection of the target antigen. Thorough specificity testing ensures that the diagnostic antibody reliably distinguishes the target antigen from other similar molecules, improving the accuracy and reliability of diagnostic assays.
Conclusion
In conclusion, optimizing Phage Display for high-performing antibody extraction requires a meticulous approach to each step of the process. From ensuring a diverse and robust phage library to employing effective selection and screening strategies, each aspect plays a crucial role in the success of antibody discovery. Enhancing antibody affinity through mutagenesis and computational design further refines the process, leading to the development of antibodies with superior binding characteristics.
Phage Display technology offers unparalleled advantages in antibody discovery. Its high throughput and versatility make it suitable for a wide range of targets, while its capability for affinity maturation allows for the continuous improvement of antibody binding properties. The diversity accessible through both synthetic and natural libraries increases the likelihood of identifying high-affinity binders, and the ability to customize antibodies for specific applications underscores the flexibility of this technology.
ProteoGenix excels in harnessing the full potential of Phage Display, providing comprehensive and customized solutions for antibody discovery. With a team of experienced scientists and cutting-edge facilities, ProteoGenix ensures high-quality results tailored to the specific needs of each project. Our expertise spans from library construction and screening to affinity maturation and validation, offering end-to-end support for your research and development endeavors.
To delve deeper into the intricacies and applications of Phage Display technology, we invite you to visit our comprehensive Phage Display Technology Guide. This resource offers udetailed information on the history, methodology, and advanced techniques used in Phage Display, providing valuable insights for researchers and scientists.
For those interested in leveraging our expertise in antibody discovery, explore our Phage Display Services. ProteoGenix offers a wide range of services, including custom phage display library construction, high-throughput screening, and antibody engineering. Our dedicated team is committed to delivering high-quality, high-affinity antibodies tailored to your specific needs.
Additionally, if you are looking to enhance the binding properties of your antibodies, our Antibody Affinity Maturation Services provide cutting-edge solutions to optimize antibody performance. Using advanced techniques such as error-prone PCR, DNA shuffling, and computational modeling, we can significantly improve the affinity and specificity of your antibodies.
We encourage you to contact ProteoGenix for collaboration or inquiries about our services. Our team of experts is ready to support your research and development projects, ensuring the successful discovery and optimization of high-performing antibodies. Partner with us to leverage our comprehensive Phage Display solutions and accelerate your scientific breakthroughs.