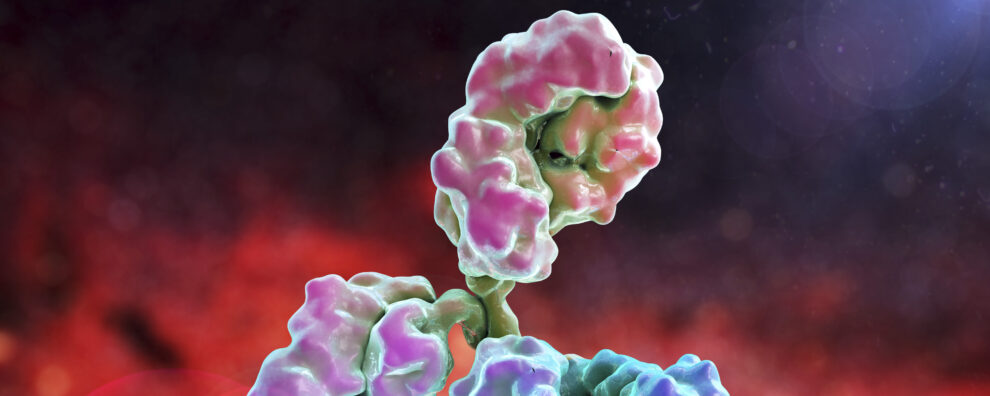
The Ultimate Guide to Phage Display Technology: Unlocking the Future of Monoclonal Antibody Development
Scientists conducting research in industries from health care and biotechnology to agricultural sciences have long required the need to identify proteins that bind their target of interest. This is a problem that has been historically difficult to solve. Let’s say you are a scientist who wants to identify a novel peptide inhibitor drug or antibody that blocks the function of a cell surface enzyme you have spent your whole career studying. You’re convinced that such a drug would revolutionize the treatment of pancreatic cancer, saving millions of lives. But there is a problem. Identifying the perfect inhibitor would involve screening tens of billions of different molecules taking several lifetimes to identify that ‘needle in a haystack’ inhibitor. What if there was a way to find this novel peptide drug or monoclonal antibody by screening billions of candidates simultaneously? A method that would find that needle in the haystack rapidly in an unbiased manner. Phage display is a biotechnological advancement that achieves this and much more. This blog post is your phage display encyclopedia to help you learn everything you need to know about phage display and more. Let’s begin.
- Brief Overview of Phage Display:
- Importance and Relevance in Modern Biotechnology:
- Purpose of the blog:
- What is Phage Display?
- Definition and Basic Concept:
- Key Terminology:
- The history of Phage Display:
- Discovery and Development of Phage Display:
- Milestones in the Evolution of the Technology:
- Principles of Phage Display
- How Does Phage Display Work:
- The Science Behind Phage Display:
- Phage Display Library Construction
- Types of Phage Display Libraries:
- Methods and Techniques for Constructing Libraries:
- Library Screening Processes:
- What Is The Difference Between An Immune Library and A Naïve Library?
- What are the Advantages and Disadvantages of Naïve and Immune Libraries?
- Applications of Phage Display
- Monoclonal Antibody Development:
- Drug Discovery and Development:
- Protein-Protein Interactions:
- Vaccine Development:
- Protein Engineering:
- Diagnostics:
- Target Validation:
- Peptide Library Screening:
- Other Emerging Applications:
- Advantages and Limitations
- Benefits of Using Phage Display Technology
- Potential Challenges and Limitations
- Which Antibody Formats Could Be Developed Through Phage Display?
- Single-Chain Variable Fragments (scFvs)
- Fab Fragments
- Bivalent and Bispecific Antibodies
- Nanobodies
- Antibody-Drug Conjugates (ADCs)
- Chimeric Antigen Receptor (CAR) T Cells
- Chimeric Autoantigen Receptor (CAAR) T Cells
- Multispecific Antibodies
- Antibody Fragments for Therapeutics and Diagnostics
- Antibody Phage Display – The Superior Method For Producing Antibody Fragments
- Frequently Asked Questions (FAQs)
- Conclusion
- Selected publications
Brief Overview of Phage Display:
Phage display is a groundbreaking laboratory technique for studying protein interactions with other molecules such as other proteins, peptides, and DNA. The method uses bacteriophages (viruses that infect bacteria) to tether the genetic code with the surface-exposed protein. Here is what that means.
Think of bacteriophages as submicroscopic packages. Inside each package are DNA molecules that encode proteins exposed to the outer surface of the package. Each package, in theory, contains a different DNA sequence that encodes a unique surface-exposed protein. The collection of bacteriophage ‘packages’ are collectively referred to as a phage display library.
Next, the phage display ‘bait’, is immobilized to capture bacteriophage ‘packages’. The bacteriophages that stick to the bait remain on the surface while other bacteriophages are washed away. The DNA inside the phages is sequenced to reveal the genetic code of the surface-exposed protein that binds the bait. And there you have it. A high-throughput, nonbiased, method for identifying ‘needle in a haystack’-proteins that bind your target of interest.
This technique allows for the screening of large combinatorial libraries, facilitating the identification of peptides, proteins, and antibodies that bind the ‘bait’ with high affinity and specificity. Therefore, phage display allows researchers to link genotype with phenotype, making phage display a versatile tool in molecular biology and biotechnology.
Importance and Relevance in Modern Biotechnology:
Phage display has become a cornerstone in modern biotechnology, thanks to the rapid identification and optimization of high-affinity binders from diverse libraries. Specifically, phage display has accelerated the following biotechnology methods:
- Therapeutic development
- Antibody identification
- Vaccine development
- Protein engineering
- Epitope mapping
- Drug discovery
- Diagnostics
This has significant implications for drug discovery, diagnostics, and therapeutic development, where precision and efficiency are paramount. Additionally, phage display technology is instrumental in mapping protein interactions and identifying novel targets for therapeutic intervention.
Purpose of the blog:
This blog aims to provide a comprehensive review of phage display technology, elucidating its principles, methodologies, and diverse applications. We will delve into the construction and screening of phage display libraries, explore the different types of libraries, and highlight the latest advancements and trends in the field. By offering detailed insights and practical knowledge, we seek to inform and educate researchers and scientists about the immense potential of phage display technology
What is Phage Display?
Definition and Basic Concept:
Phage display is a molecular technique that involves the expression of peptides, proteins, or antibody fragments on the surface of bacteriophages—viruses that infect bacteria. This method enables high-throughput screening of large combinatorial libraries to identify high-affinity binders. The process begins by inserting a gene encoding a protein or peptide of interest into the phage genome, leading to the display of the encoded protein on the phage’s outer surface. Researchers can then screen these phage-displayed proteins against target molecules to isolate those with the highest affinity and specificity. Phage display technology has revolutionized various fields, including drug discovery, diagnostics, and therapeutic antibody development. By enabling the rapid screening of vast molecular libraries, phage display accelerates the identification and optimization of high-affinity interactions crucial for developing new therapeutics and diagnostic tools.
Key Terminology:
- Bacteriophage (Phage): A virus that infects bacteria. In phage display, filamentous phages like M13, fd, and f1 are commonly used due to their ability to display foreign peptides on their surface.
- Library: A diverse collection of phage particles, each displaying a different peptide or protein variant. These libraries can contain billions of unique sequences, allowing extensive screening capabilities. Libraries can be derived from natural sources or synthesized to include specific sequences of interest.
- Panning (Biopanning): The selection process where phage-displayed peptides or proteins are screened for binding to a specific target molecule. This involves incubating the phage library with the target, washing away non-binders, and amplifying the bound phages for further rounds of selection.
- Affinity Maturation: The process of evolving proteins or antibodies to enhance their binding affinity to a target through iterative rounds of mutation and selection. Techniques such as site-directed mutagenesis and chain shuffling are often employed to achieve this.
- Genotype-Phenotype Linkage: The direct connection between the genetic material (DNA) encoding a displayed peptide or protein and its corresponding phenotype (the displayed molecule on the phage surface). This linkage facilitates the identification and recovery of high-affinity binders.
The history of Phage Display:
Discovery and Development of Phage Display:
Phage display technology was first conceptualized and demonstrated by George P. Smith in 1985. Smith’s pioneering work involved the fusion of peptide sequences to the coat protein of filamentous bacteriophage, allowing peptides to be displayed on the surface of the phage particles. This innovation enabled the linkage of the displayed peptide (phenotype) with its encoding genetic material (genotype), facilitating the identification and isolation of peptides with specific binding properties. Smith’s initial experiments used the filamentous phage M13 to display foreign peptides as fusions with the minor coat protein pIII. This groundbreaking approach laid the foundation for subsequent developments in phage display technology, enabling the display of a wide range of peptides and proteins on the surface of bacteriophages.
Milestones in the Evolution of the Technology:
- 1985: George P. Smith publishes his seminal work on the display of peptides on the surface of filamentous bacteriophage, introducing the concept of phage display.
- 1990: John McCafferty, Andrew D. Griffiths, Greg Winter, and David J. Chiswell extend phage display technology to the display of antibody fragments. They demonstrate the display of functional antibody fragments (Fabs) on the surface of filamentous phage, opening new avenues for antibody discovery and engineering.
- 1991: The first antibody fragment displayed on phage is selected against a target antigen, showcasing the potential of phage display for antibody discovery and leading to the development of recombinant antibody libraries.
- 1994: The creation of large combinatorial libraries displaying billions of different peptides or proteins marks a significant advancement, greatly enhancing the screening capabilities of phage display technology.
- 1996: The approval of the first phage display-derived therapeutic antibody, adalimumab (Humira®), by the FDA. Adalimumab, a fully human monoclonal antibody targeting TNFα, exemplifies the clinical potential of phage display technology.
- 2002: The development of synthetic antibody libraries, which incorporate synthetic sequences to create highly diverse libraries with predefined characteristics, further expands the utility and flexibility of phage display.
- 2018: The Nobel Prize in Chemistry is awarded to George P. Smith and Sir Gregory P. Winter for their work on the phage display of peptides and antibodies, recognizing the profound impact of this technology on the field of biotechnology and medicine.
Phage display technology has continued to evolve, with advancements in library design, selection strategies, and antigen presentation methods. These improvements have enhanced the efficiency and specificity of the technology, enabling the discovery of antibodies and peptides with high affinity and novel functionalities. Today, phage display is a cornerstone of modern biotechnology, widely used in drug discovery, diagnostics, and therapeutic development.
Principles of Phage Display
How Does Phage Display Work:
Phage display is a technique where a peptide or protein is genetically fused to a coat protein of a bacteriophage, such that the peptide or protein is displayed on the exterior surface of the phage while the genetic material encoding the protein resides within the phage particle. This allows a direct linkage between the phenotype (displayed protein) and the genotype (encoding DNA). The general workflow of phage display involves the following steps:
- Library Construction: A diverse library of phage particles is created, each displaying a different peptide or protein variant on its surface. This library can be constructed using a variety of methods, including random peptide libraries, cDNA libraries, or synthetic libraries.
- Panning (Selection): The phage library is exposed to an immobilized target (e.g., a protein, cell, or small molecule). Phages that display peptides or proteins with a high affinity for the target bind to it, while non-binders are washed away. Bound phages are then eluted and amplified by infecting E. coli bacteria.
- Amplification: The eluted phages are amplified by infecting a bacterial host, typically E. coli. This allows for the production of a large quantity of the selected phage, which can then undergo additional rounds of panning to further enrich for high-affinity binders.
- Screening and Characterization: After several rounds of panning and amplification, individual phage clones are isolated and characterized for their binding properties. This can include sequencing the DNA to identify the displayed peptides or proteins, as well as binding assays to determine affinity and specificity.
The Science Behind Phage Display:
Phage display leverages the biology of bacteriophages and their interactions with bacterial hosts. Key scientific principles include:
- Genotype-Phenotype Linkage: By displaying a peptide or protein on the surface of the phage and maintaining the corresponding DNA inside the phage, there is a direct linkage between the physical traits (phenotype) and the genetic information (genotype). This allows for the easy identification of binding sequences.
- Selection Pressure: The iterative process of binding, washing, eluting, and amplifying introduces a selection pressure that enriches for phages displaying peptides or proteins with the highest affinity for the target. This mimics natural selection on a molecular level, allowing for the evolution of high-affinity binders.
- Library Diversity: The success of phage display relies heavily on the diversity of the library. Larger libraries increase the probability of finding high-affinity binders. Libraries can be constructed from natural sources or synthetically, incorporating a wide range of peptide or protein sequences.
- Affinity Maturation: Through repeated rounds of selection and amplification, phage display can be used to perform affinity maturation, where the binding affinity of selected peptides or proteins is progressively increased. This is analogous to the natural immune response, where antibodies undergo somatic hypermutation and selection for higher affinity binding.
Phage Display Library Construction
Types of Phage Display Libraries:
Phage display libraries are essential tools in biomedical research and drug development, and they can be broadly categorized into peptide libraries and antibody libraries.
- Peptide Libraries: These libraries consist of a diverse array of short peptides displayed on the surface of bacteriophages. Peptide libraries are utilized to map protein-protein interactions, identify epitopes, and discover peptide ligands that bind to specific target molecules. The diversity in peptide libraries is generated by synthesizing random peptide sequences and inserting them into the phage genome. Learn more about our custom peptide synthesis and peptide libraries.
- Antibody Libraries: Antibody libraries display antibody fragments, such as single-chain variable fragments (scFvs) or Fab fragments, on the phage surface. These libraries are crucial for discovering and optimizing monoclonal antibodies. Antibody libraries can be classified into several types:
- Naïve Libraries: Derived from the natural immune repertoires of non-immunized donors, providing a broad diversity of antibody sequences that can bind to a wide range of antigens. Explore our naïve antibody library services.
- Immune Libraries: Generated from the B cells of immunized donors, these libraries contain high-affinity antibodies that have undergone somatic hypermutation in response to a specific antigen. They are particularly useful for isolating high-affinity antibodies against challenging targets. Learn more about our immune library services.
- Synthetic Libraries: Constructed using synthetic oligonucleotides to create a highly diverse pool of antibody sequences. These libraries can be designed with specific characteristics and are not limited by the natural immune repertoire.
- Semisynthetic Libraries: Combine elements of both natural and synthetic libraries, using natural antibody frameworks with synthetic diversity introduced into the complementarity-determining regions (CDRs). This approach leverages the structural integrity of natural antibodies while enhancing diversity and specificity.
Methods and Techniques for Constructing Libraries:
The construction of phage display libraries involves several key steps to ensure high diversity and functionality:
- Source Material: For peptide libraries, synthetic oligonucleotides create a diverse pool of peptide sequences. For antibody libraries, genetic material encoding the variable regions of antibodies is obtained from immune cells. This can include naïve, immune, or synthetic repertoires, each contributing to different aspects of the library’s diversity and functionality. Learn more about our antibody library services.
- Insertion into Phage Genome: The synthesized or amplified DNA sequences are inserted into the phage genome at a location that allows the encoded peptides or proteins to be displayed on the phage coat protein, typically the pIII or pVIII protein in filamentous phages like M13.
- Transformation and Amplification: The recombinant phage DNA is introduced into a bacterial host, such as E. coli, through a process called electroporation. The transformed bacteria produce phage particles displaying the diverse library of peptides or proteins on their surfaces. The phage particles are then collected and amplified to create a large, diverse library.
- Quality Control and Validation: The constructed library is subjected to quality control measures to ensure the diversity and functionality of the displayed sequences. This includes sequencing a subset of the library to verify the presence of diverse and correctly inserted sequences.
Library Screening Processes:
Phage display library screening involves several iterative steps to enrich for phages that display peptides or proteins with high affinity for a target molecule:
- Panning (Selection): The phage library is exposed to an immobilized target. Phages displaying peptides or proteins that bind to the target are retained, while non-binders are washed away. This process, known as panning, is repeated multiple times to enrich for high-affinity binders.
- Elution and Amplification: The bound phages are eluted from the target and amplified by infecting a bacterial host. This selective amplification increases the proportion of phages displaying high-affinity binders in the library.
- Screening: After several rounds of panning and amplification, individual phage clones are isolated and characterized for their binding properties. Techniques such as ELISA (enzyme-linked immunosorbent assay) and SPR (surface plasmon resonance) are used to determine the affinity and specificity of the selected peptides or proteins.
- Sequencing: The DNA encoding the displayed peptides or proteins from high-affinity phages is sequenced to identify the binding sequences. This genetic information can be used for further optimization and development of the identified binders.
What Is The Difference Between An Immune Library and A Naïve Library?
An immune library is a type of antibody phage display library where the antibody-producing B cells are isolated from an immunized host. Immune libraries therefore contain a heterogenous pool of antibodies that have high affinity toward a particular antigen. The high antibody is a consequence of affinity maturation where B cells or plasma cells have undergone affinity maturation and selection in vivo.
Naive antibody phage display libraries are built from a pool of antibodies that have not been exposed to vaccine antigens and therefore have not undergone any selection or affinity maturation processes. These libraries can be made from humans since naïve libraries do not require host vaccination.
Both naïve and immune antibody phage display libraries can be engineered into different antibody formats by designing PCR primers that bind conserved regions in the desired immunoglobulin heavy or light chains.
What are the Advantages and Disadvantages of Naïve and Immune Libraries?
Naïve antibody phage display libraries are quicker and cheaper to build compared to immune libraries. Many antibody production companies like ProteoGenix have a diverse collection of pre-built naïve libraries from a range of species and antibody formats.
What immune libraries lack in speed and cost they make up for in precision. Below are the differences between naïve and immune antibody phage display libraries.
Naive Phage Display Library | Immune Phage Display Library | |
---|---|---|
Diversity | High diversity | Limited diversity |
Affinity Maturation | No | Yes |
Specifity | +++ | + |
Library generation time | +++ | ++ |
Applications of Phage Display
The numerous applications of phage display technology, in its simplest form, involve identifying proteins that interact with a homogenous immobilized molecule, the bait, with a heterogenous pool of proteins located on the surface of bacteriophages.
Specifically, phage display technology can indiscriminately identify the DNA sequence of proteins, such as small peptide chains or whole antibodies, that bind to any molecule you can dream of with incredible sensitivity. This is a game-changing technology that has revolutionized biomedical science, biotechnology, health care, and the agricultural industry.
This powerful technique has been utilized in various applications, ranging from drug discovery and development to antibody engineering and vaccine design. Let’s explore the wide range of applications where phage display technology.
Monoclonal Antibody Development:
Phage display technology has revolutionized monoclonal antibody development by allowing the rapid and efficient identification of high-affinity antibodies. This method involves creating vast libraries of antibody fragments, such as single-chain variable fragments (scFvs) or Fab fragments, displayed on the surface of bacteriophages. These libraries are screened against various antigens to isolate antibodies with desired specificities and affinities. Notable monoclonal antibodies discovered using phage display include:
- Adalimumab (Humira®): The first fully human monoclonal antibody approved for therapeutic use, targeting TNFα for treating autoimmune diseases like rheumatoid arthritis.
- Panitumumab (Vectibix®): A fully human monoclonal antibody targeting the epidermal growth factor receptor (EGFR) for the treatment of colorectal cancer.
Phage display has also facilitated the engineering of antibodies with enhanced properties such as improved stability, reduced immunogenicity, and enhanced developability, making it a cornerstone in modern therapeutic antibody discovery and development.
Drug Discovery and Development:
Phage display is a critical tool in drug discovery, particularly for identifying peptide and protein ligands that modulate the activity of disease-associated targets. The technology allows for high-throughput screening of phage libraries to identify peptides that bind with high affinity and specificity to target proteins, providing valuable leads for drug development. Key applications in drug discovery include:
- Enzyme Inhibitors: Identifying peptides that inhibit enzyme activity, which can serve as leads for developing new therapeutic agents.
- Receptor Ligands: Discovering peptides that can act as agonists or antagonists of cell surface receptors, influencing signaling pathways involved in various diseases.
The ability to perform iterative rounds of selection and optimization through phage display significantly enhances the efficiency of identifying and refining lead compounds for therapeutic development.
Protein-Protein Interactions:
Understanding protein-protein interactions is crucial for elucidating the molecular mechanisms underlying various biological processes and diseases. Phage display technology is widely used to study these interactions by selecting peptides or antibody fragments that bind to specific protein targets. Applications in protein-protein interactions include:
- Mapping Interaction Networks: Identifying peptides that bind to known protein partners, helping to map out interaction networks within cells.
- Functional Domain Identification: Discovering peptides that bind to specific functional domains within proteins, providing insights into their roles in cellular processes.
By dissecting these interactions at a molecular level, phage display provides critical information that can be used for drug development and therapeutic intervention.
Vaccine Development:
Phage display has made significant contributions to vaccine development by enabling the identification of immunogenic epitopes. These epitopes can be displayed on the surface of bacteriophages to create phage-based vaccines that elicit strong immune responses. Applications in vaccine development include:
- Viral Vaccines: Developing vaccines against viral pathogens by displaying viral epitopes on phages, which can induce protective immunity.
- Bacterial Vaccines: Identifying bacterial epitopes that can be used to create vaccines against bacterial infections.
Phage display-derived peptides and proteins are also used as diagnostic tools for detecting immune responses to pathogens, aiding in the development of more effective and safe vaccines.
Protein Engineering:
Phage display allows for the generation of protein variants with altered properties, such as increased stability or binding affinity, which can be useful for developing improved biologics or enzymes. This fascinating phage display application involves making a phage display library with random DNA mutations introduced into the genetic code of a protein of interest. Next, the library would undergo stress such as high temperature, followed by identifying which variants retained the ability to bind its endogenous ligand (the bait).
Diagnostics:
Phage display can assist in the identification of biomarkers for various diseases, enabling the development of diagnostic tests for early detection and monitoring of conditions. For example, phage libraries displaying human brain proteins have been used to identify patients who present with symptoms of autoimmune encephalitis, a condition where autoantibodies attack the human brain. Here is how it works. The patients who test negative for all brain autoantibodies known to science submit a blood sample to identify and later diagnose, novel autoantibodies causing rare forms of autoimmune encephalitis.
Target Validation:
Phage display libraries can be screened against cellular antigens to validate therapeutics. For instance, a newly identified peptide inhibitor can be immobilized and used as bait to identify proteins from cells or tissues that bind to the peptide inhibitor. This approach can also identify undesirable cross-reactive targets of the newly-developed drug.
Peptide Library Screening:
By displaying random peptide sequences on phages, researchers can screen large libraries for peptides with desired functions, such as receptor ligands or enzyme inhibitors. In this example, the enzyme or receptor is used as bait to identify which peptides the immobilized target interacts with. The DNA encoding the novel peptides can later be used for drug development.
Other Emerging Applications:
Phage display technology continues to find new applications across various fields of biotechnology and medicine. Some of the emerging applications include:
- Biosensor Development: Phage display-derived peptides and proteins are used to develop biosensors for detecting environmental pollutants, pathogens, and biomarkers. These biosensors offer high sensitivity and specificity, making them valuable tools for environmental monitoring and diagnostics.
- Gene Therapy: Phage display can be used to select peptides and proteins that enhance the delivery of gene therapy vectors to target cells, improving the efficiency and specificity of gene transfer.
- Cancer Therapy: Phage display is being explored for developing targeted cancer therapies, including antibody-drug conjugates (ADCs) and chimeric antigen receptor (CAR) T-cell therapies. These approaches leverage phage display-derived antibodies to specifically target cancer cells while sparing normal tissues.
Phage display technology continues to evolve with advancements in library design, selection strategies, and applications. As the technology matures, it is expected to play an increasingly important role in developing new therapeutics, diagnostics, and research tools.
Advantages and Limitations
Benefits of Using Phage Display Technology
- High Diversity and Specificity:
Library Construction: Phage display technology enables the construction of highly diverse libraries of peptides, proteins, and antibody fragments. This diversity allows for the identification of molecules with high specificity and affinity for a wide range of targets, including proteins, cells, and small molecules.
Customizability: The technology allows for the creation of custom libraries tailored to specific needs, such as human, synthetic, and immune libraries. This flexibility makes it possible to target a variety of antigens and discover unique binding sequences.
- Speed and Efficiency:
Rapid Screening: Phage display accelerates the screening process by allowing high-throughput selection of binding molecules. This is particularly advantageous in drug discovery and therapeutic antibody development, where time is a critical factor.
In Vitro Selection: The entire process can be performed in vitro, eliminating the need for animal models and reducing the time and resources required for discovery and development.
- Broad Applications:
Therapeutic Antibodies: Phage display has been instrumental in developing numerous therapeutic antibodies, such as adalimumab (Humira®) and trastuzumab (Herceptin®), which are used to treat various diseases, including cancer and autoimmune disorders.
Drug Discovery: The technology is widely used to discover peptide and protein ligands that can modulate the activity of therapeutic targets, facilitating the development of novel drugs.
Vaccine Development: Phage display enables the identification of immunogenic epitopes for vaccine development, aiding in the design of effective and safe vaccines.
- Molecular Evolution and Engineering:
Affinity Maturation: Phage display allows for the in vitro evolution of molecules through iterative rounds of selection and mutation, leading to the development of high-affinity binders. This process is crucial for optimizing therapeutic antibodies and other biologics.
Functional Studies: The technology is used to study protein-protein interactions, identify functional domains, and understand the molecular basis of binding, providing valuable insights for drug development and therapeutic interventions.
Potential Challenges and Limitations
- Complexity of Library Construction:
Technical Expertise: Constructing high-quality phage display libraries requires significant technical expertise and resources. The process involves cloning, transformation, and amplification, which can be technically demanding and time-consuming.
Quality Control: Ensuring the diversity and functionality of the library is critical. Poor library quality can lead to reduced success rates in identifying high-affinity binders, necessitating rigorous quality control measures.
- Selection Bias:
Target Presentation: The success of phage display depends on how the target is presented during the panning process. Improper presentation can lead to selection bias, where only certain types of binders are enriched, potentially missing other high-affinity candidates.
Non-Specific Binding: Phage particles can sometimes bind non-specifically to targets or selection surfaces, complicating the identification of true high-affinity binders. This requires careful optimization of selection conditions to minimize non-specific interactions.
- Post-Selection Validation:
Functional Testing: After selecting binders, extensive validation is required to confirm their binding affinity and specificity in functional assays. This step is critical to ensure that the selected molecules are effective in their intended applications.
Downstream Development: Transitioning from phage-displayed binders to fully functional therapeutic molecules involves additional engineering, expression, and purification steps, which can be challenging and resource-intensive.
- Intellectual Property and Licensing:
Patent Landscape: The extensive use of phage display technology in various fields has led to a complex patent landscape. Researchers and companies must navigate intellectual property rights and licensing agreements, which can be restrictive and costly.
- Freedom to Operate: Ensuring freedom to operate is essential for commercial development, requiring careful consideration of existing patents and potential infringement issues.
Which Antibody Formats Could Be Developed Through Phage Display?
Single-Chain Variable Fragments (scFvs)
Single-chain variable fragments (scFvs) are fusion proteins of the variable heavy (VH) and variable light (VL) chains linked by a short linker peptide and not actually fragments of an antibody. This format retains the antigen-binding specificity of full-length antibodies while being smaller and more stable.
Applications in Research and Therapeutics: scFvs are widely used in research for mapping epitopes and studying protein interactions. In therapeutics, scFvs are utilized in targeted cancer therapies, where their small size allows for better tissue penetration and rapid clearance from the bloodstream, reducing potential side effects.
Fab Fragments
Fragment antigen binding region (Fab) is the part of the antibody that physically interacts with antigens. They consist of one constant and one variable domain from each of the heavy and light chains of an antibody. They retain full antigen-binding capability but lack the Fc region, making them smaller and less prone to inducing immune responses.
Therapeutic and Diagnostic Applications: Fab fragments are used in therapeutic settings where full-length antibodies are not required, such as in the treatment of macular degeneration (e.g., ranibizumab) or as antivenoms for rattlesnake bites (Digoxin immune Fab and Crofab). These antibody fragments are smaller, easier to penetrate tissues, and are less likely to trigger anaphylaxis because the conserved, non-human, Fc region is removed1. They are also employed in diagnostic assays due to their high specificity and stability.
Bivalent and Bispecific Antibodies
Natural antibodies are bivalent meaning they have two identical antigen-binding sites, while bispecific antibodies are artificial antibodies that can simultaneously bind two different epitopes on the same antigen or from different antigens. These formats are engineered by linking different scFvs or Fabs, often using phage display libraries to select the optimal binders.
Therapeutic Applications: Bivalent and bispecific antibodies are particularly valuable in cancer immunotherapy, where they can bring immune cells into proximity with cancer cells, enhancing the immune response against tumors. They are also used in targeting multiple pathways simultaneously to improve therapeutic outcomes. Currently, three bispecific antibodies are being used in clinical settings. Blinatumomab is utilized to target CD19 and CD3 for the treatment of acute lymphoblastic leukemia (ALL). Emicizumab neutralizes clotting factors IXa and X to treat hemophilia A. Lastly, Amivantamab is a licensed bispecific antibody that is used to treat metastatic non-small cell lung cancer by targeting epidermal growth factor and MET receptors.
Nanobodies
Nanobodies, or single-domain antibodies (sdAb) an antibody fragments consisting of a single variable domain. With a molecular mass of 12-15 kDa, nanobodies are less than 10% of the mass of human IgG antibodies.
The first single-domain nanobodies were engineered from camelids, referred to as VHH fragments, known for their small, stable, and tissue-penetrating properties compared to other mammalian antibodies. Cartilaginous fish, such as sharks, naturally express exceptionally small antibodies immunoglobulin new age receptor (IgNAR) antibodies. These antibodies can be expressed as even smaller monomeric chains called variable new age receptor (VNAR) fragments for enhanced tissue penetration in vivo.
Applications in Imaging, Research, and Therapeutics: Due to their small size and stability, nanobodies are used in molecular imaging to track disease progression in real time. They are also valuable in research for studying protein interactions and as therapeutic agents for conditions like neurodegenerative diseases and cancer.
Antibody-Drug Conjugates (ADCs)
Antibody-drug conjugates (ADCs) are a class of biological drugs used as cancer immunotherapies. ADCs designed to direct cytotoxic chemical cargo to directly kill tumor cells, unlike chemotherapy which can kill healthy tissues. The antigen-binding region directs the conjugated drug to specific cells, while the cytotoxic agent kills the targeted cells.
Phage display is used to identify high-affinity antibodies specific to tumor-associated antigens, which are then conjugated to cytotoxic drugs to create effective ADCs.
ADCs are currently used in the treatment of various cancers, including breast cancer (e.g., trastuzumab emtansine). Research is ongoing to improve the stability, efficacy, and safety profiles of ADCs.
Chimeric Antigen Receptor (CAR) T Cells
Role of Phage Display in CAR T cell Development: CAR T cells are engineered T-cells expressing a chimeric antigen receptor that give T cells the new ability to destroy an antigen-specific target cell such as cancer cells. Phage display is used to identify high-affinity antibodies that serve as the binding domain for CAR constructs.
Applications in Cancer Immunotherapy: CAR T cell therapy has shown remarkable success in treating certain types of cancer, such as B cell lymphomas and acute lymphoblastic leukemia (ALL). The ability to target specific cancer cells while sparing normal cells makes this a powerful therapeutic approach.
Chimeric Autoantigen Receptor (CAAR) T Cells
Phage Display in CAAR Development: CAAR T cells are similar to CAR T cells but instead of being engineered to detect a single antigen expressed on a tumor cell, the T cell expresses a decoy antigen that binds the B cell receptor (BCR), a cell surface-bound immunoglobulin expressed by mature, autoantibody-producing, B-cells. This feature allows T cells the ability to seek and destroy harmful memory B cells, curing the autoimmune condition. Peptide phage display libraries can be used to identify peptide candidates well suited for binding autoreactive BCRs from pathogenic memory B cells.
Multispecific Antibodies
Multispecific antibodies, also known as bispecific and trispecific antibodies, are engineered to bind multiple epitopes on the same antigen or different target antigens simultaneously, providing broader therapeutic effects. Phage display is a powerful approach to facilitate the identification and optimization of these antibodies to ensure effective binding and functional properties.
Multispecific antibodies are being developed for complex diseases that require targeting multiple pathways, such as cancer and autoimmune diseases. These antibodies offer the potential to enhance therapeutic efficacy and reduce drug resistance.
Antibody Fragments for Therapeutics and Diagnostics
Use of Smaller Antibody Fragments: Smaller antibody fragments, such as Fabs, scFvs, and nanobodies, are developed for specific therapeutic and diagnostic applications. Their small size enhances solubility while increasing tissue penetration, stability, and ease of production, making them ideal for certain clinical settings.
Development Through Phage Display: Phage display is a key technology for screening and optimizing these fragments, ensuring high specificity and affinity for their targets.
Future Prospects for Therapeutic and Diagnostic Applications: Ongoing research aims to enhance the stability, reduce immunogenicity, and improve the delivery of these antibody fragments to target tissues. Their versatility makes them valuable tools in both therapeutic and diagnostic applications.
Antibody Phage Display – The Superior Method For Producing Antibody Fragments
Traditional Methods for Creating Antibody Fragments: Non-phage display methods involve cloning the desired section of the monoclonal antibody using polymerase chain reaction (PCR) and inserting the fragment into an expression vector. The expression vector is transfected into cells so it can be converted into antibody fragments that are purified and tested for antigen-binding potential.
This process is time-consuming and tedious process has a major flaw. There is no way of knowing if the fragment will retain the desired antigen affinity, solubility, or stability of the intended application.
The solution is to isolate a series of antibody fragment candidates from an antibody fragment library with high affinity to the target antigen. This increases the odds of identifying at least one high-performing antibody fragment with the desired characteristics.
Frequently Asked Questions (FAQs)
- How is Phage Display used in vaccine development? Phage display is an innovative technique employed in vaccine development to identify and select immunogenic epitopes, which can be displayed on the surface of bacteriophages to create phage-based vaccines that elicit strong immune responses. The process begins with the construction of diverse peptide libraries by inserting a wide array of peptide or protein sequences from various pathogens into the phage genome, allowing for the creation of vast libraries with billions of different sequences. During the panning (selection) process, the phage library is exposed to antibodies or immune cells from vaccinated or infected individuals, leading to the selective binding of phages displaying recognized epitopes. These bound phages are then isolated, amplified, and subjected to further rounds of selection to enrich for high-affinity binders, ensuring the identification of epitopes with the highest immunogenic potential. Following selection, the epitopes are mapped and characterized through sequencing and various assays to determine their effectiveness in eliciting an immune response. The identified immunogenic epitopes are then used to design phage-based vaccines, which can be engineered to display multiple epitopes, enhancing their ability to stimulate a broad and robust immune response. The phage particles themselves can also act as adjuvants, further boosting the immune response. Preclinical testing of these vaccines in animal models evaluates their safety, immunogenicity, and efficacy, followed by clinical trials in humans to assess their potential as effective vaccines against infectious diseases. This comprehensive approach ensures that phage display technology plays a crucial role in developing vaccines that are both effective and versatile.
- Can Phage Display be used to study protein-protein interactions? Yes, phage display is widely used to study protein-protein interactions by selecting peptides or antibody fragments that bind to specific protein targets, helping to map interaction networks and identify functional domains within proteins. This method leverages the ability to link the phenotype (displayed peptide or protein) with the genotype (encoding DNA), allowing researchers to efficiently identify and characterize protein interactions. Phage display libraries, displaying diverse peptides or proteins, are screened against target proteins through an iterative selection process called panning, which enriches for high-affinity binders. The binding sequences of selected phages are then sequenced, providing insights into interaction sites and functional domains, crucial for understanding the molecular mechanisms of protein function. This technique has been successfully applied to various protein interaction studies, offering high throughput and specificity, and is valuable for mapping protein interaction networks and discovering interaction inhibitors with therapeutic potential.
- What are some emerging trends in Phage Display technology? Emerging trends in phage display technology include the integration of next-generation sequencing (NGS) for comprehensive library analysis, the use of CRISPR-Cas9 for enhanced library construction, and the development of novel phage display formats and screening techniques. NGS allows for high-throughput and detailed analysis of phage display libraries, providing insights into the diversity and evolution of selected binders. CRISPR-Cas9 technology is utilized to create more diverse and precisely edited libraries, improving the efficiency and specificity of phage display selections. Additionally, advancements in phage display formats, such as using different bacteriophages and engineering phage coat proteins, enhance the display capabilities and expand the range of potential applications. These innovations are driving the evolution of phage display technology, making it a more powerful tool for drug discovery, diagnostics, and therapeutic development.
- What are the challenges of Phage Display? Phage display technology faces several challenges, including managing the complexity of constructing high-quality libraries, addressing potential selection biases, ensuring rigorous validation of binders, and navigating the intellectual property landscape. Constructing high-quality phage display libraries requires significant expertise and resources, as it involves generating diverse and functional peptide or antibody sequences. Selection biases can occur during the panning process, where non-specific binders or phages with growth advantages may be preferentially selected, complicating the identification of true high-affinity binders. Rigorous validation of selected binders is essential to confirm their specificity and functionality, often necessitating additional in vitro and in vivo testing. Additionally, the extensive use of phage display in various fields has led to a complex patent landscape, requiring careful navigation of intellectual property rights and licensing agreements to ensure freedom to operate and commercial development.
- Can Phage Display be used to develop therapeutic antibodies? Yes, phage display is a key technology in developing therapeutic antibodies, allowing for the rapid identification and optimization of antibodies with high specificity and affinity for target antigens. This technique involves creating large libraries of antibody fragments, such as single-chain variable fragments (scFvs) or Fab fragments, displayed on the surface of bacteriophages. These libraries are screened against specific antigens to select high-affinity binders.Phage display facilitates the selection process by linking the phenotype (the displayed antibody fragment) with the genotype (the encoding DNA). Through iterative rounds of binding, washing, and amplification, high-affinity antibodies are enriched and identified. This method is particularly advantageous for developing fully human antibodies, reducing the risk of immunogenicity when used in patients. The application of phage display in therapeutic antibody development has led to significant advancements. For example, adalimumab (Humira®), the first fully human monoclonal antibody approved for therapeutic use, was developed using phage display technology. This antibody targets TNFα and is used to treat autoimmune diseases such as rheumatoid arthritis. Phage display also allows for the rapid optimization of antibodies, enhancing their affinity, specificity, and stability. This is achieved through techniques such as affinity maturation, where mutations are introduced into the antibody genes, and high-affinity variants are selected.
- How is Phage Display different from other display technologies? Phage display offers unique advantages like the ability to perform selections in vitro, the ease of linking phenotype and genotype, and the capability to create highly diverse libraries, distinguishing it from other display technologies like yeast display or ribosome display. Phage display is robust and well-established, utilizing bacteriophages to display peptides or antibody fragments on their surface, which allows for efficient screening and identification of high-affinity binders in a controlled in vitro environment. This technology directly links the displayed peptide or protein (phenotype) with its encoding DNA (genotype), facilitating the rapid identification and sequencing of selected binders. In contrast, yeast display utilizes the eukaryotic yeast system to express proteins on the surface of yeast cells, providing a more native-like environment for eukaryotic proteins and offering advantages in the expression of complex proteins. However, yeast display can be more complex and time-consuming due to the need for yeast cell culture and maintenance. Ribosome display is a cell-free system that involves the formation of stable mRNA-ribosome-protein complexes, allowing for the selection of high-affinity binders without the need for transformation into living cells. This method can generate highly diverse libraries and avoid potential biases introduced by cellular systems, but it requires specialized equipment and can be technically challenging to perform. Phage display’s simplicity, versatility, and high-throughput capabilities make it a preferred choice for many applications, including antibody discovery, peptide selection, and protein engineering. Its ability to perform selections in vitro and directly link genotype to phenotype distinguishes it from yeast and ribosome display technologies, offering unique advantages in various research and therapeutic contexts.
- What Is The Difference Between a pIII and pVIII phage display libraries? Antibodies, proteins, or peptides can be fused to either pIII or pVIII phage coat proteins. However, there are advantages and disadvantages of each. The first difference between pIII and pVIII libraries is the concentration of surface-exposed proteins displayed on the surface of the phage. For example, there are only 3 to 5 pIII proteins on the surface of the bacteriophage, while there are roughly 3,000 pVIII proteins. Therefore, pVIII libraries display proteins on the bacteriophage surface more densely than pIII libraries increasing the binding potential with the immobilized ‘bait’. The pIII gene encodes a nonfunctional protein whereas pVIII is required to infect bacteria. Therefore, pVIII libraries typically only contain short peptides of 6-7 residues that exclude cysteine, required to preserve pVIII function. This means antibody phage display libraries are engineered using pIII libraries. The choice between pIII and pVIII fusions can affect the selection process, with pIII being suitable for higher-affinity ligands and pVIII allowing for the selection of low-affinity ligands due to the increased avidity effect of high valency display.
Conclusion
Summary of Key Points: Phage display is a revolutionary technology that enables the study of protein interactions, the development of monoclonal antibodies, and the discovery of novel therapeutic agents. This technique involves displaying peptides, proteins, or antibody fragments on the surface of bacteriophages, facilitating the high-throughput screening of large combinatorial libraries. Phage display has a wide array of applications, including drug discovery, vaccine development, and the study of protein-protein interactions. The integration of next-generation sequencing and CRISPR-Cas9 technologies has further enhanced the efficiency and precision of phage display, making it an indispensable tool in modern biotechnology.
Final Thoughts on the Importance of Phage Display: Phage display technology has profoundly impacted the fields of molecular biology and biotechnology. Its ability to link phenotype to genotype allows for rapid and efficient identification of high-affinity binders, which is crucial for the development of targeted therapies. Phage display continues to drive innovation in therapeutic antibody development, personalized medicine, and diagnostics. As advancements in this technology continue to emerge, its applications and potential are expanding, promising even greater contributions to science and medicine.
To learn more about how phage display can accelerate your research and development projects, visit our Phage Display Services page. Our comprehensive services include antibody library construction, screening, and optimization to meet your specific needs. For more information, feel free to contact us directly. Our team of experts is ready to assist you in harnessing the power of phage display technology to achieve your scientific goals.