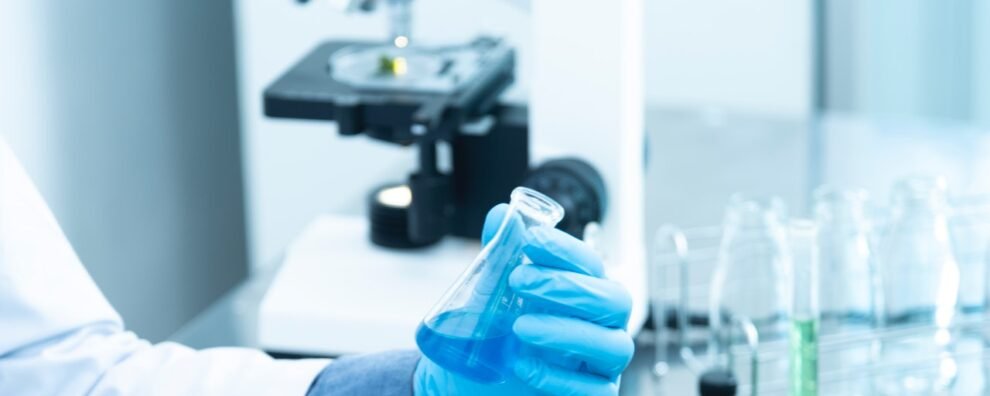
Peptide-based biomaterials for tissue engineering
In recent years, the use of biomaterials for tissue engineering has been gaining ground over conventional grafting for repairing damaged tissue. These biomaterials are often a combination of stable polymeric support modified with peptides or proteins designed to foster tissue repair. In this article, we explore the advantages of using easily synthesized peptides over complex and hard to produce proteins. Additionally, we go over the most promising peptide-based biomaterials for tissue repair applications.
Tissue engineering versus conventional grafting
Tissue engineering is a promising alternative approach to conventional grafting. Grafts are surgical procedures designed to move tissue from one site to another or from a donor to a patient, replacing damaged skin, bone, cartilage, nerves, or vessels with healthy ones. In contrast, biomaterials for tissue engineering comprise scaffolds, cells, and bioactive molecules. This synergistic combination is currently one of the best and safest options for tissue repair and regeneration.
The typical elements that comprise biomaterials for tissue engineering include:
- Scaffolds: composites, or natural and synthetic polymers
- Bioactive molecules: small molecules, proteins, or peptides such as cell adhesion peptides and self-assembling peptides
- Cells (optional): mesenchymal stem cells (MSCs)
The interest in this field has been steadily growing due to the increase in chronic disease incidence, the low availability of donors for conventional grafting, and the inherent limitations of medical transplants. The objective of these biomaterials is to foster tissue regeneration in patients, either using cell-free supports modified with bioactive molecules or a combination of these modified scaffolds with the patients’ own MSCs.
Scaffolds and cells used in biomaterials for tissue engineering
Designed to mimic the natural extracellular matrix, scaffolds are biocompatible, biodegradable, porous, and stable structures able to support cellular growth. Natural polymers used for these applications include collagen, chitosan, elastin, and hyaluronic acid (HA), among others. Synthetic polymers used as scaffolds in biomaterials for tissue engineering typically include poly-ε-caprolactone (PCL), polylactic acid (PLA), and poly-glycolic acid (PGA), among others.
Both natural and synthetic polymers have specific advantages and limitations. For instance, natural polymers are known for their high biocompatibility but poor mechanical properties, often leading to premature breakdown. In contrast, synthetic polymers tend to be more stable than their natural counterparts but exhibit low biocompatibility, often leading to inflammation and, ultimately, rejection of the biomaterial. Enhancing the biocompatibility mitigates the risk of adverse reactions and is often achieved by modifying the surface of scaffolds, natural or synthetic, with bioactive molecules.
Cells are another vital component of these biomaterials, isolated from adipose tissue or bone marrow, adult mesenchymal stem cells (MSCs) are currently the gold standard for regenerative medicine. They exhibit the ability to self-regenerate and differentiate into different cell types, making them useful building blocks for new and healthy tissue.
Bioactive molecules used in biomaterials for tissue engineering
Among the plethora of biomolecules currently used in biomaterials for tissue engineering, peptides play a vital role as modifiers of polymeric scaffolds. When compared to biomaterials modified with proteins, peptide-modified scaffolds exhibit similar properties. But in contrast to proteins, the use of peptides for tissue applications offers several significant advantages:
- Peptide synthesis is much simpler and cost-effective than protein expression
- Peptide modification is straightforward
- These small molecules are also more resistant to environmental conditions such as temperature and pH, whilst proteins tend to degrade in non-physiological conditions
- Peptides tend to have lower immunogenicity in comparison to large proteins
For this reason, peptides have become the preferred bioactive component of biomaterials for tissue engineering applications. Some examples of peptides with outstanding bioactivity are provided in the table below:
Type of peptides | Origin | AA sequence/name of the bioactive motif |
---|---|---|
Cell adhesion peptides | Collagen | RGD |
DGEA | ||
GFOGER | ||
GFPGER | ||
GTPGPQGIAGQRGVV (PepGen P-15) | ||
Fibronectin | PHSRN | |
REDV | ||
LDV | ||
KQAGDV | ||
Laminins | IKVAV | |
YIGSR | ||
KAFDITYVRLKF | ||
Bone morphogenetic protein (BMP)-like peptides | BMP-2 | IVAPPGYHAFYCHGECP (P17) |
KIPKASSVPTELSAISTLYLSGGC (P24) | ||
BMP-7 | GQGFSYPYKAVFSTQ (BFP-1) | |
KQLNAISVLYFDD | ||
Vascular endothelial growth factor (VEGF)-like peptides | VEGF | KLTWQELYQLKYKGI (QK) |
Self-assembling peptides (SAPs) | Synthetic | RADARADARADARADA (RAD16) |
QQRFEWEFEQQ (P11) | ||
QQKFQFQFEQQ (Q11) | ||
VKVKVKVKVdPLPTKVKVKVKV-NH2 (MAX1) | ||
VKVKVKVKVdPLPTKVEVKVKV-NH2 (MAX8) |
All these classes of peptides enhance the biocompatibility of the biomaterials used for tissue regeneration. More specifically, cell adhesion peptides improve cell adhesion, proliferation, and differentiation. In contrast, VEGF-like and BMP-like peptides mimic these proteins by controlling vessel and bone repair, respectively.
Interestingly, self-assembled peptides merit a section of their own. These peptides form complex three-dimensional structures that self-assemble spontaneously via non-covalent bonds (i.e., hydrogen bonds, electrostatic and hydrophobic interactions). The process can be triggered by external signals such as pH, temperature, or chemical molecules.
Like the other classes of peptides, SAPs can be easily produced via solid-phase peptide synthesis. Moreover, the formation of complex nanostructures can take place under physiological conditions, making the process inexpensive and highly efficient. These structures, also called peptide hydrogels, can themselves mimic the extracellular matrix and serve as scaffolds for tissue-regenerating biomaterials.
Self-assembling peptides as scaffolds for tissue engineering
Self-assembling peptides are a rapidly-evolving class of fully synthetic peptides, yet they consist of entirely natural building blocks. According to experts, peptide hydrogels represent one of the most promising elements for a variety of applications. They remain biocompatible despite their synthetic nature with safe degradability profiles and minimal immunogenicity. Moreover, hydrogels can be easily manipulated to obtain scaffolds with different physical properties such as pore size, fiber thickness, and mechanical resistance.
RAD-based peptides remain one of the most used biomaterials for tissue engineering. These peptides are based on the RAD sequence, a 16 amino acid sequence, developed by Zhang and co-workers at the MIT. RAD16 gelation can be induced in the presence of salt (even at low concentrations) or low pH values. In this hydrogel state, cells are able to attach to the surface of RAD16 matrices, making it invaluable as a scaffold for tissue-repairing biomaterials. Although the first generation of RAD16 peptides is already commercially available, their use for biological applications is only now starting to be explored. The new generation of RAD19 biomaterials has been progressively modified with active and natural bioactive motifs to enhance tissue regeneration and biocompatibility.
P11 peptides comprise another fully synthetic class of self-assembling bioactive molecules. These hydrogelating peptides assemble in response to hydrophobic interactions, which can drive their rearrangement into fibrils and fibers. Some peptides of this class only assemble in response to the addition of a chemical counterpart, making them amenable to in situ assembly.
Despite the promising results obtained in preclinical and clinical studies, self-assembly peptides can remain challenging to synthesize and purify. The issues arise from the presence of multiple hydrophobic residues within the sequence of hydrogels. Another issue is the potential contamination with TFA (trifluoroacetic acid), particularly when these peptides are purified using high-performance liquid chromatography (HPLC). TFA is known to negatively affect cell proliferation, warranting its removal via lyophilization in the presence of hydrochloric acid or via resin-ion exchange.
Concluding remarks
The development of peptide-based biomaterials for tissue engineering applications is a dynamic and rapidly-evolving field of study. These materials are poised to replace conventional grafts due to their cost-effective and straightforward production process.
- Klimek, K. and Ginalska, G. Proteins and Peptides as Important Modifiers of the Polymer Scaffolds for Tissue Engineering Applications—A Review. Polymers (Basel). 2020; 12(4): 844. doi: 10.3390/polym12040844
- Maude, S. et al. Biomimetic self-assembling peptides as scaffolds for soft tissue engineering. Nanomedicine (Lond). 2013; 8(5):823-847. doi: 10.2217/nnm.13.65
- Zhang, S. et al. Spontaneous assembly of a self-complementary oligopeptide to form a stable macroscopic membrane. Proc Natl Acad Sci U S A. 1993; 90(8):3334-3338. doi: 10.1073/pnas.90.8.3334