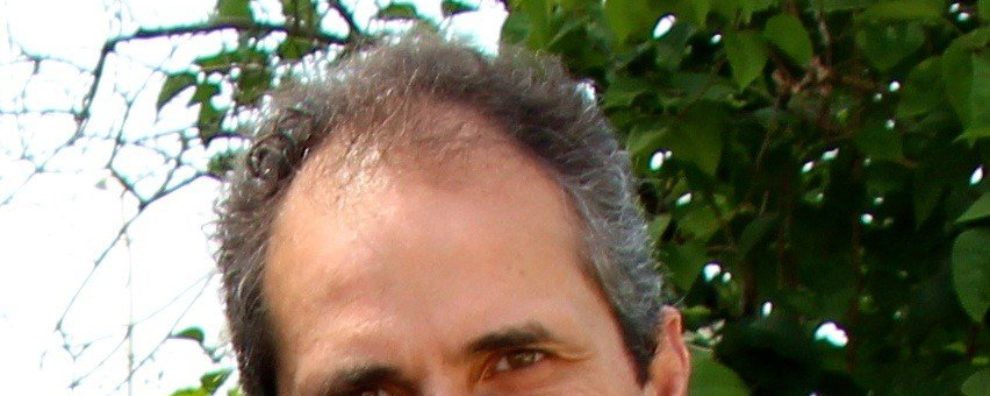
“We are living in an exciting time for the field of antibody and gene therapy”
Dr. Bernard Schneider has devoted his career to the development of exciting gene and antibody delivery technologies to stop the progression of neurodegeneration. Recently, he and his team at the École Polytechnique Fédérale de Lausanne (EPFL, Switzerland) have developed a bioimplant able to deliver antibodies in a constant and renewable way, alleviating the need to produce large amounts of these complex biomolecules or to submit patients to frequent injections. We talked with Dr. Schneider about the challenges and breakthroughs he has experienced throughout his exciting career in the Neurosciences.
- ProteoGenix: Can you tell us about your academic and professional journey? What has inspired you to devote your career to the neurosciences and the study of neurodegenerative diseases?
- In which areas of research is the EPFL focused at the moment?
- In your scientific career, what are the greatest challenges you and colleagues have faced in the fight against neurodegeneration?
- You talked about the difficulties in understanding what to target when fighting the progression of these diseases. Do you think these targets are only disease-specific or also population-specific? Do you think they’ll be different from person to person?
- How do you think we can overcome these problems; could they solved by including more genomics and proteomics analysis in the study of these diseases?
- From a more specific point of view, what are the greatest challenges when developing therapeutic antibodies for the treatment of Central Nervous System (CNS) diseases?
- In the context of therapeutic antibodies, in a recent study, you have proposed the use of bioactive antibody-secreting implants to suppress the development of Alzheimer’s. How would this treatment work?
- Hence, from a practical point of view, the ideal location of this implant would be the brain?
- What type of cells have you considered using for these implants?
- Is it easy to encapsulate these cells? And what type of materials have you considered for this process?
- This approach for the treatment of neurodegenerative diseases has several advantages. In your opinion, what are the most critical obstacles for its clinical application?
- Until now, what do you consider to be the most exciting results you and your team obtained with this approach?
- Tell us more about other exciting projects that are happening in your lab.
- What do you think will be the greatest breakthrough in neurodegenerative therapy in the coming decades?
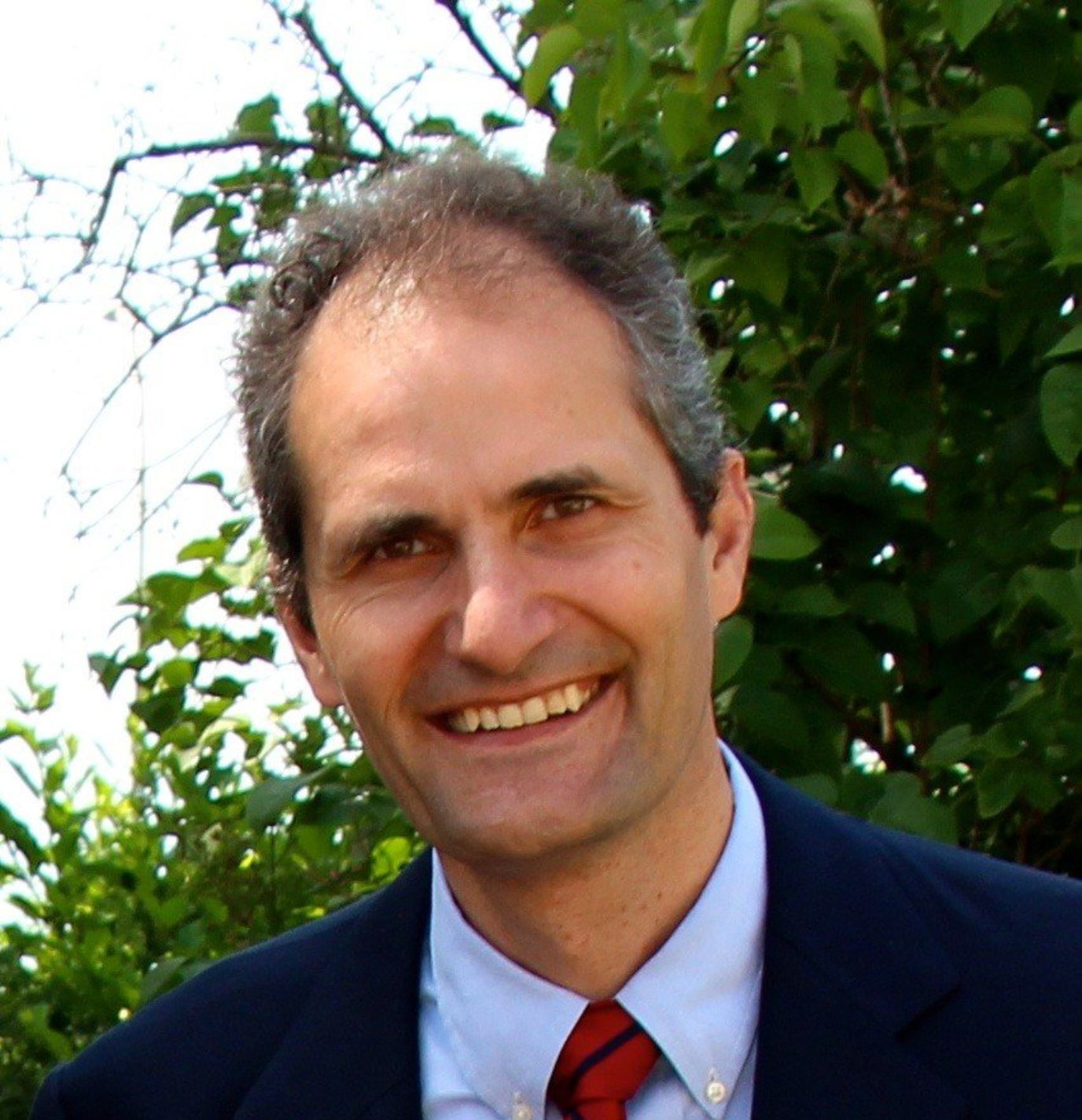
ProteoGenix: Can you tell us about your academic and professional journey? What has inspired you to devote your career to the neurosciences and the study of neurodegenerative diseases?
Dr. Bernard Schneider: What sparked my interest in the neurosciences was a mix of two things. The first was a deep curiosity in understanding how the brain and neurons work. And the second one relates to the type of research that resonates with me on a personal level. I’m the kind of scientist who likes to think about applications and to see a practical purpose in our scientific endeavors.
The mix of these two interests led me to study neurodegeneration and to get involved in the development of therapeutic applications that could target these diseases in the early and later stages.
This also led me to the EPFL in Lausanne, where I decided to pursue my academic career. As you know, EPFL is one of two schools of technology here in Switzerland, and it is essentially devoted to applications and to the development of technology that answers the needs of our society. Here, I have the opportunity to develop an academic career aligned with my expectations.
In which areas of research is the EPFL focused at the moment?
EPFL is focused on developing new technologies to treat cancer, neurodegeneration, and infectious disorders. These key areas show you that the school doesn’t exactly work like a conventional University. Instead, it is more devoted to the development of specific applications and that is what motivates me to continue developing my research here.
In your scientific career, what are the greatest challenges you and colleagues have faced in the fight against neurodegeneration?
We continuously face two challenges. The first one is caused by our still poor understanding of what is causing the progressive degeneration of the brain at an abnormal speed. Today, we understand some of the main mechanisms involved in these processes, many of these are based on genetic defects. However, for the majority of cases, which occur sporadically, we still have a poor understanding of what is causing these diseases.
This uncertainty regarding the causes, also makes it harder and more complex to think about clinical applications because, in most instances, we have to adapt our target to what we know about the disease.
The second aspect relates more to the brain, which, by itself is an organ that is complex and difficult to target. Often, it is extremely difficult to translate a mechanism or a strategy on how we could interfere with the disease into an effective and feasible application. This difficult translation from the bench to the patients happens due to inherent constraints of the blood-brain barrier, to the size of the brain itself, or even to the need to define targets from a population of neurons or cells in a very specific manner.
I think this is where our current tools are still limited.
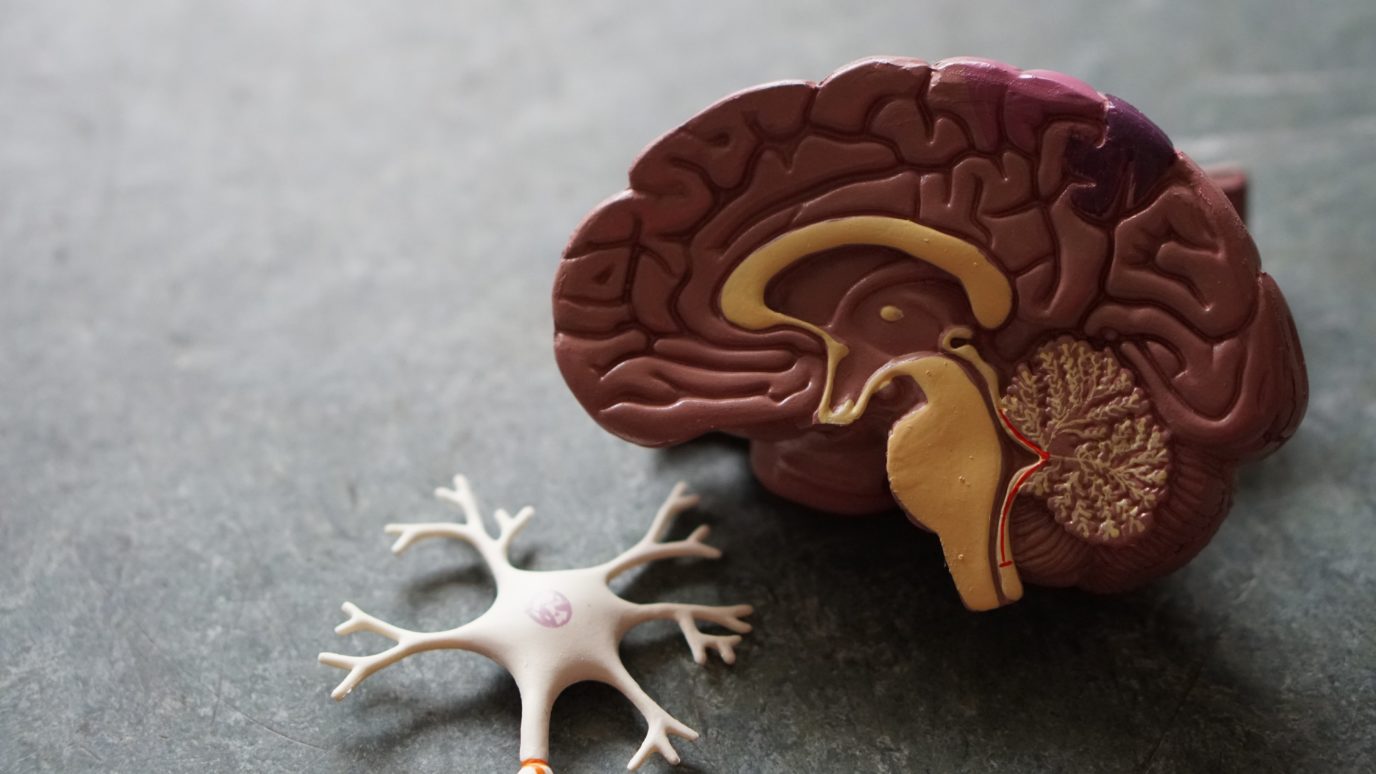
You talked about the difficulties in understanding what to target when fighting the progression of these diseases. Do you think these targets are only disease-specific or also population-specific? Do you think they’ll be different from person to person?
We’re all hoping to find a general target, but the real picture is much more complex than that. There are differences between patients and diseases. There are also differences depending on the stage of progression of the disease. If you think about Alzheimer’s for instance, what may be applicable in the early stages may no longer be applicable in later stages of the disease.
Moreover, we also still have a relatively poor ability to stratify different populations of patients with different needs, which also creates some problems in our ability to design effective therapies.
How do you think we can overcome these problems; could they solved by including more genomics and proteomics analysis in the study of these diseases?
We could at least start to understand them by including all the battery of possible analysis for the detection of disease markers in the brain – specifically through brain imaging – combined with the search for biomarkers that could be present in the CSF (Cerebrospinal Fluid), or other biofluids such as the blood that would be more easily accessible.
But timing is perhaps the most important factor we need to be able to master to guarantee the success of any therapeutic approach. We need to understand when to start the treatment, under which conditions and in which type of patients will a certain treatment be most effective. Which is also tied to our ability to detect the disease at an early stage and to monitor its subsequent progression.
From a more specific point of view, what are the greatest challenges when developing therapeutic antibodies for the treatment of Central Nervous System (CNS) diseases?
Typically, the antibodies we have been developing target the proteinopathies related to the observed deposition of misfolded forms of α-synuclein, tau, or amyloid-β (Aβ). The difficulty here is that we are aiming at a moving target.
These proteins are frequently changing conformation and distribution in the brain. It is believed that the disease is associated with the transition from protein monomers, towards oligomers or aggregates. Along this path, proteins often undergo extensive post-translational modifications, such as in the case of tau. Thus, it’s difficult to have a very clear definition of what structure of the target we will need to translate to an in vitro or animal model for our studies. In the end, this interferes with our ability to construct faithful replicates of the human pathology.
Secondly, neurodegeneration is a dynamic process and research keeps extending the potential diversity of targets. For instance, research on post-translational modifications and their role at different stages of the diseases has increased target diversity in the last decades.
Moreover, considering the different protein conformations we can find in the brain, further expands on potential target diversity. When we consider differences in conformation, we’re not only thinking about single types of fibrils or single types of oligomers, but also about protein strains which could be derived from the same protein in the beginning, but may acquire different conformations depending on the disease or even the patient.
In the context of neurodegeneration, the misfolding and aggregation of these protein markers is considered to be the central mechanism that fuels the progression of these diseases. But we are still missing a very clear identification of the precise toxic species, and also, we are not absolutely certain about which part of the process is toxic, or if some cellular compartments are more important than others. In summary, we still have a very incomplete picture.
And I think that shows you how difficult it is to identify which antibody can do the job. Antibodies are interesting tools to interfere with the pathogenic process, this is clear, but we need to also be much more precise in our understanding of what it is we need to target in disease pathogenesis.
In the context of therapeutic antibodies, in a recent study, you have proposed the use of bioactive antibody-secreting implants to suppress the development of Alzheimer’s. How would this treatment work?
The idea behind this study was to develop cellular implants that would provide a continuous renewable source of antibodies. We have worked with different formats of bioimplants, shapes, inner volume, and with the idea of either delivering the antibody in the periphery or directly inside the brain.
The latter case provides a clear advantage as it allows us to deliver the antibody directly on site. This approach would overcome the hurdle caused by the brain-blood barrier, which is the strongest limitation to the development of immunotherapies targeting neurodegeneration. The limitation could also be partially solved by injecting extremely high doses of antibodies in the periphery, but this would become very costly when considered at a large-scale.
Thus, we came up with the concept of bioimplants to bypass these hurdles. This mode of delivery would be constant, which also comes with certain advantages in terms of pharmacokinetics, and potentially easier to apply at an early stage of the disease. Bioimplants would also reduce the need for frequent injections, therefore alleviating the need for patients to come to the clinic regularly.
Hence, from a practical point of view, the ideal location of this implant would be the brain?
We considered both the brain and the periphery. The concept of implanting a capsule containing cells inside the brain is not new. We have been developing and optimizing this technology in our labs for a long time, which we first applied to neurotrophic factors and then adapted for antibody-delivery.
On-site delivery provides many advantages in terms of pharmacokinetics and it allows us to quickly achieve therapeutic dosages without having to expose the patient to high antibody concentrations.
But in the most recent study, we also investigated the possibility of implanting the capsules in the periphery. With this, we wanted to test if it was possible to avoid the need to perform brain surgery for intracerebral device implantation. This approach, however, also comes with several very specific challenges. For instance, when we consider peripheric implants we need to devise capsules able to produce much higher doses of antibodies than the ones produced by capsules we can implant in the brain.
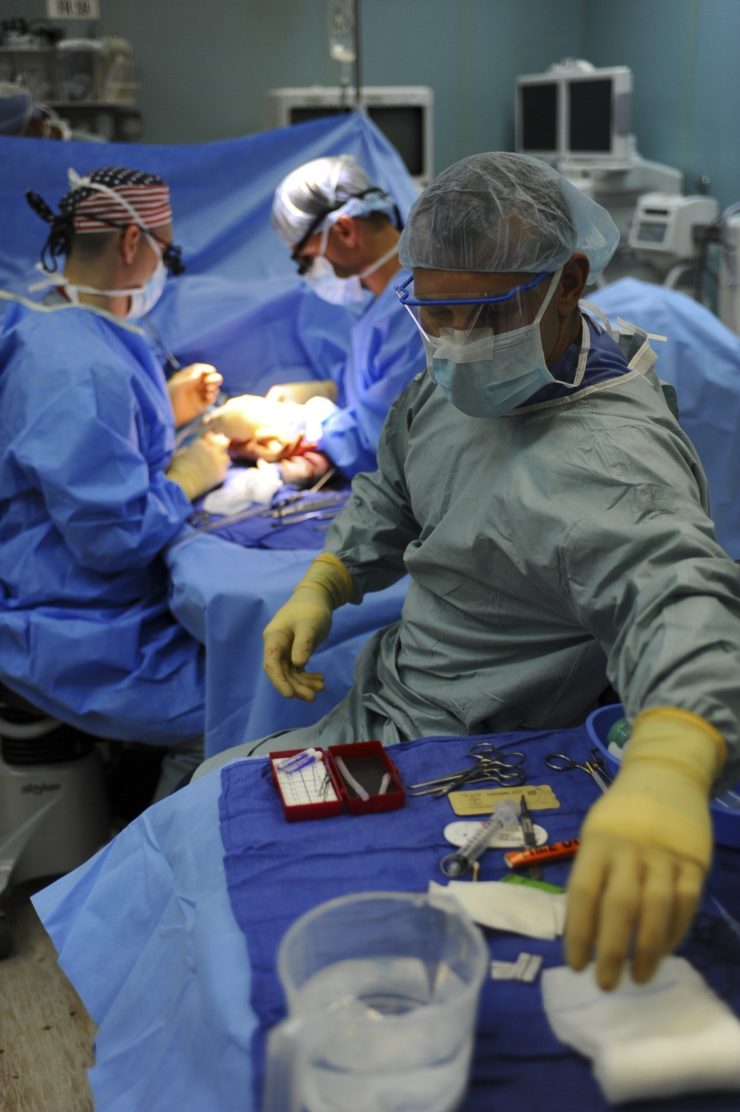
What type of cells have you considered using for these implants?
This aspect of our concept is in constant development. We came up with the idea that myoblasts would be an interesting cell type for two reasons, the first is that they adapt quite well to the inherent metabolic limitations inside the capsule. Myoblasts are dividing cells which can differentiate into myotubes that maintain a good capability to secrete recombinant proteins such as antibodies. This makes them promising candidates for cell encapsulation.
But, the one difficulty about working with myoblasts is that you don’t have easily accessible sources of myoblasts of human origin. In the case of the implantation of the capsule we need to use cells of allogenic origin: so, when we work with mice, we need to use mouse cells, but when we consider applications in humans, we need to use human cells. Therefore, the need for good sources of human myoblasts became an obvious constraint in the development of our technology.
Another constraint is the immortalization of these cells. Now there are better strategies to immortalize human myoblasts that maintain their differentiating capabilities into myotubes.
Despite these shortcomings, I think myoblasts are a very good cell type for this type of application. For this reason, it’s also urgent that we focus our efforts on the development of a renewable source of human myoblasts compatible with cell encapsulation and with its use in the clinic.
Is it easy to encapsulate these cells? And what type of materials have you considered for this process?
The main difficulty here is not to introduce the cells into the capsules. This process can be done in a very easy way by a simple injection procedure, with the extra volume of medium ultrafiltrating through the permeable membrane. The difficulty relates more to creating a niche where the cells can freely grow in a three-dimensional manner. This niche also requires that we find and use a suitable substrate to support cell growth.
We have used a lot of different technologies in the past. But we obtained the most interesting results with hydrogels. Hydrogels provide a degradable support for myoblasts and allow them to achieve a 3D structure. And their use is now further expanding the possibilities to develop encapsulating systems with large inner volumes.
This approach for the treatment of neurodegenerative diseases has several advantages. In your opinion, what are the most critical obstacles for its clinical application?
Number one is the need for good antibodies. We need antibodies with demonstrated efficacy in targeting neurodegenerative diseases in humans. Unfortunately, we are still only at the very beginning of that search.
In the context of Alzheimer’s disease, we may now have the first indications that something exciting and promising is about to happen. After so many failures over many years, there is a first glimpse of a significant effect, but the future will tell.
Besides good antibodies, another main challenge is the ability to achieve a stable and constant dosage. To control the dosage of antibodies secreted by our bioimplants we need to be able to predict their behavior through time. Ideally, the final dosing will be determined by the inner volume of the capsules.
Moreover, we need to be able to ensure that the implant secretes dosages able to produce a therapeutic effect. Scale-wise this has proven to be a great challenge for translation, considering the obvious difference in size between mice and humans.
We believe our approach will also benefit from improvements in current antibody technologies. If we can generate antibodies that are more selective, stable, able to pass the blood-brain barrier or antibodies that have other features that maybe I cannot even imagine yet, we will be able to develop more effective bioimplants.
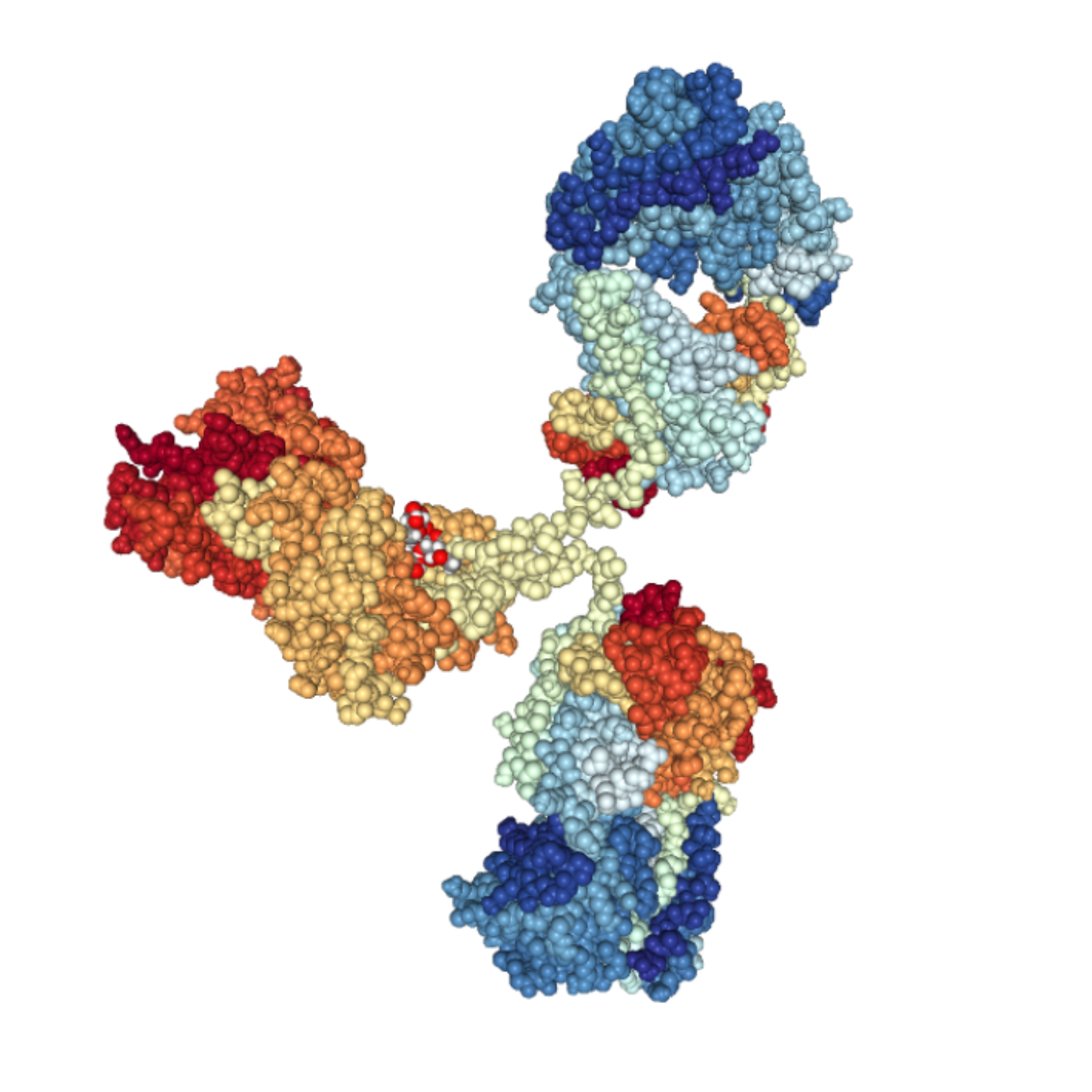
Until now, what do you consider to be the most exciting results you and your team obtained with this approach?
Due to the limitations of conventional mouse models, we have also focused our efforts on their refinement to better assess the efficacy of our technology. Specifically, we are working to combine the Aβ and the tau pathology into a single model in an attempt to better mimic the human disease. With these models, we still see that, even when delivered during the later stages of the disease, the antibody-secreting bioimplants have a marked effect on some aspects of these pathologies, and not on others.
Moreover, our results suggest that these bioimplants might also be able to manipulate the activity of microglia cells, which are one of the key players in the development of Alzheimer’s disease.
Up to recently, our understanding of how microglia interferes with plaques in presence of the tau pathology was limited. And the reason for this is that we had been trying to consider these actors linearly. This is well illustrated by the amyloid-β hypothesis, which states that the amyloid-β causes tau aggregation and inflammation, which, in turn, would evolve into a full disease spectrum.
Now it’s becoming more and more evident that, instead of a linear process, it is likely that microglia activity, tau pathology and Aβ pathology act in concert and together lead to the development of the disease.
The fact that an antibody implanted into the periphery or into the brain can bind amyloid plaques and interfere with this complex pathogenic environment, is the most exciting result we obtained so far. This effect shows that an antibody could be an effective way to interfere with that process even when delivered through a capsule. Plus, I think that the fact that we can continuously interfere with disease progression by overcoming the shortcomings of repeated administrations, which is also something very exciting from a clinical point of view.
Tell us more about other exciting projects that are happening in your lab.
Our lab is currently evolving into a platform dedicated to gene therapy technologies. The platform will move to Campus Biotech (EPFL) in Geneva, which is dedicated to translational neuroscience.
At this moment, we are very interested in viral technologies, which are quickly developing as therapeutic tools in the context of neurological disorders. Plus, viral vectors and particles have shown great promise for the treatment of motor neuron disorders, and this will be an important research line we will be pursuing in our lab.
In the future, we hope to be able to interfere with these devastating disorders.
I believe that one way to achieve this will be to combine emerging technologies. The use of gene therapy to deliver recombinant antibodies is one such example. Another way would be to develop on-site antibody gene therapy using viral vectors, with demonstrated efficiency in the treatment of neurological disorders.
We are living in an exciting time for the field of antibody and gene therapy. And I believe we will soon see some positive outcomes for all our efforts of the last 20 years, and I’m happy to be part of it with our lab activities.
What do you think will be the greatest breakthrough in neurodegenerative therapy in the coming decades?
The goal now is not only to interfere with the symptomatic effect of the disease but also to interfere with the progression of the disease.
We now need to see the next generation of treatments able to block the cause of the disease. That would allow us to stop the progression of degeneration even during the later stages of the disease.
That will probably be something similar to what cancer has experienced recently, a combination of treatments and technologies for a more efficient approach. These technologies could comprise the use of antibodies, trophic factors, gene therapies, among others.
Of course, we still don’t know exactly what it the cause of the disease but we have some interesting targets in mind. In the context of Alzheimer’s, for instance, the real picture is much more complex than we have initially anticipated. But we already know that, in patients with very well-defined genetic causes, there are opportunities to design effective treatments.
I also expect to see even more breakthroughs soon, similar to what we have seen for the treatment of spinal muscular atrophy recently, where gene therapies are now able to change the course of the disease.
- Lathuilière, A. and Schneider, B. Un implant bioactif pour prévenir la maladie d’Alzheimer. Med Sci (Paris). 33(1): 81–84. doi: 10.1051/medsci/20173301013
- Lathuilière, A. and Schneider, B. Lentiviral Vectors for the Engineering of Implantable Cells Secreting Recombinant Antibodies. Methods Mol Biol. 2016; 1448:139-155. doi: 10.1007/978-1-4939-3753-0_11
- Lathuilière, A. et al. A subcutaneous cellular implant for passive immunization against amyloid-β reduces brain amyloid and tau pathologies. Brain. 2016; 139(Pt 5):1587-1604. doi: 10.1093/brain/aww036
- Lathuilière, A. et al. Encapsulated cellular implants for recombinant protein delivery and therapeutic modulation of the immune system. Int J Mol Sci. 2015; 16(5):10578-10600. doi: 10.3390/ijms160510578